I had the good fortune to have a conversation with Jason Crawford, from Roots of Progress, a fantastic initiative about understanding the patterns of technological evolution. Its newsletter—which I follow avidly—includes great articles such as Why did we wait so long for the bicycle?
In the book he’s writing, one chapter covers the technological evolution of energy and how it has impacted mankind, so I asked him about that. This is our conversation, edited for clarity.
The premium article this week will have the recording of the chat, as well as the last 15 minutes of our conversation, about the future of governance. I also share some of my thoughts on the entire conversation. It goes out this Thursday.
Tomas Pueyo: Energy is one of the big drivers of GDP per capita growth in history. But this has not been the case in mature economies. Is this normal in history? Is this something to be expected? How is this increase in efficiency driving or hindering our growth?
Jason Crawford: It is not normal in the grand scheme of things to have an economy grow, period. Certainly not on a per capita basis. Income per capita was basically stagnant for thousands of years. And it was only with the industrial revolution that we broke out of this Malthusian world. The challenge of the pre-industrial world was that, when you got a little bit more productive capacity, it increased the population, but not the per capita wealth.
This is the regime of Thomas Malthus: more people just sort of eat up all of the excess productive capacity. So it wasn't until around the industrial revolution timeframe that the productive capacity was growing faster than population, and actually population and per capita wealth were growing at the same time. Those things used to be in opposition: as we got more people, we'd get poorer.
Now, we have more people and we're getting richer at the same time. That is very unusual. In the last few hundred years, as this has been happening, our consumption of energy per capita has grown as well.
Just for the United States, since about 1800, per capita energy usage has been growing at, I think, around 2% per year. And of course the population itself has been growing, so the overall energy has been growing even faster than that.
In his book Where Is My Flying Car, the author J Storrs Hall pointed out that this phenomenon of increasing per capita energy usage was mentioned in the autobiography of Henry Adams. And so he calls it the Henry Adams curve.
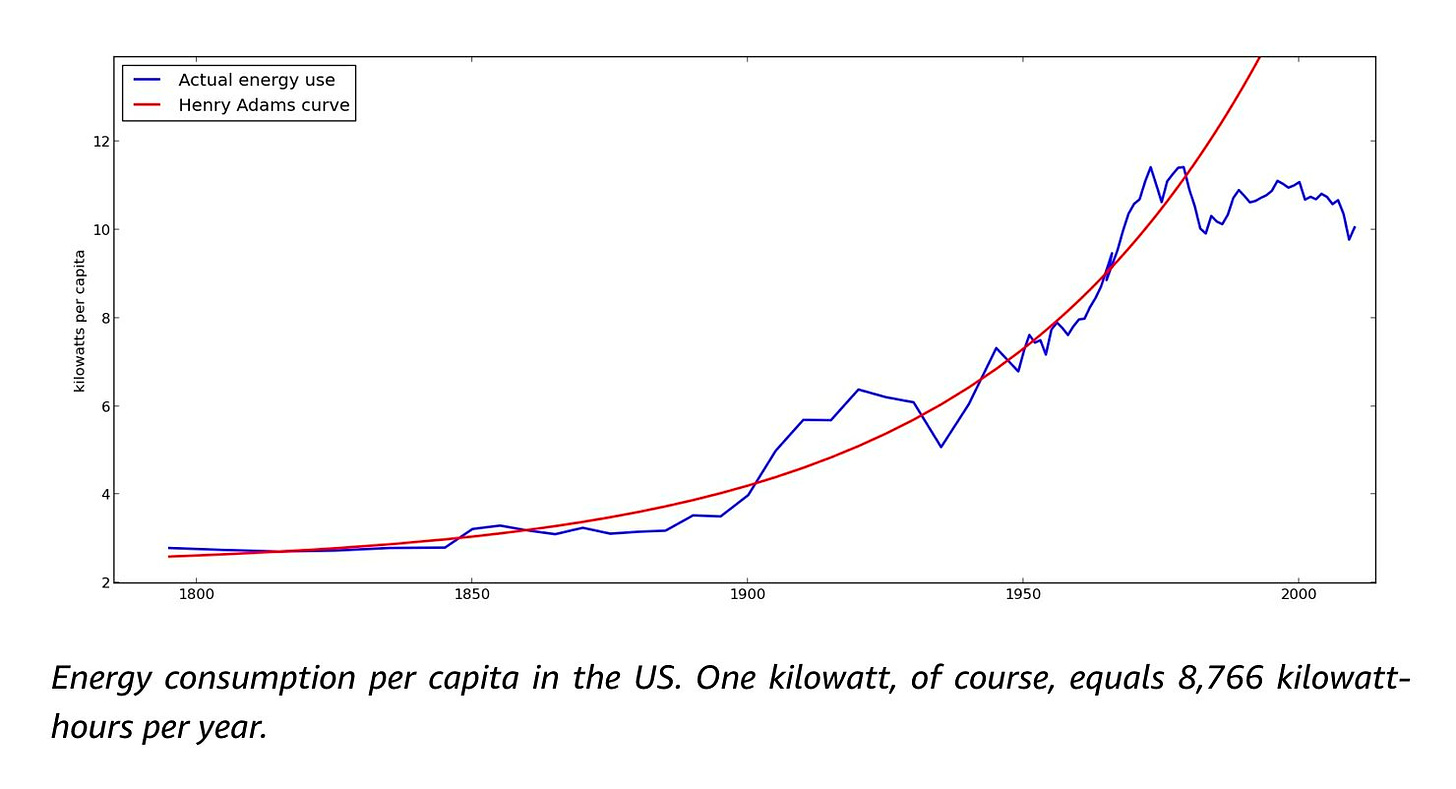
We fell off the Henry Adams curve around 1970: in the US, per capita energy usage flatlined around then and has been relatively stagnant until now. Yet in that time, GDP has continued to grow. The decoupling of these things—per capita growth going up while energy per capita is stagnant—is a historical anomaly.
Different people look at this in different ways. Some people say: “This is great! We're getting more from less. We're just becoming more efficient.” There's an implication that maybe it could go on indefinitely.
I'm highly skeptical. I think this is a short-term phenomenon. I think we will hit a wall, where at a certain point we just can't do much more in terms of increasing production without increasing both total energy usage and also energy densities, which have not increased very much. Energy is just too fundamental to the economy.
Just to briefly review:
Energy powers our factories, where we produce virtually all the goods that we use in our lives.
Energy powers material processes, such as smelting of metals or the synthesis of fertilizers or other chemicals, etc.
Energy powers our agricultural equipment, the combined harvesters that do the work of a hundred field laborers to reap grain.
Energy powers transportation, moving both cargo and people all around the world. Transportation progress has been very closely linked to energy progress and especially to the power to weight ratio of engines as that has improved throughout the decades and the centuries.
Of course, energy also powers our computing and communications infrastructure, and for advanced applications like AI and crypto, we're going to need more and more energy.
All of these things need more and more energy.
You can still see that across the world: energy usage is very closely linked to the economy. There's a tight correlation between per capita energy usage and per capita GDP.
And the relationship is reciprocal. Energy helps people be more productive by powering all those things I just mentioned. And then, conversely, when people get more income, they want to spend it on energy and on the things that are made from energy, which is essentially everything.
Another one of Hall's ideas in that book, Where's My Flying Car, is what folks like Peter Thiel and Tyler Cowen have called the Great Stagnation: a stagnating technological economy. We've gotten a lot of progress in information and communications, technology and computers and the Internet. But we've gotten relatively slower progress in transportation, manufacturing, construction, and so forth.
One hypothesis to explain it: what Thiel calls “bits versus atoms”. But I think getting a little closer to what might be one of the major causal factors is that information technology is the only technology in the last 50 years that has not required much greater energy density in order to make progress.
If we cut off the future of energy—which I think we did by stunting nuclear power back in the sixties and seventies—then this is what you would expect: we continue to make progress in the non-energy-intensive world of bits, while we stop making progress in the more energy-intensive world of atoms.
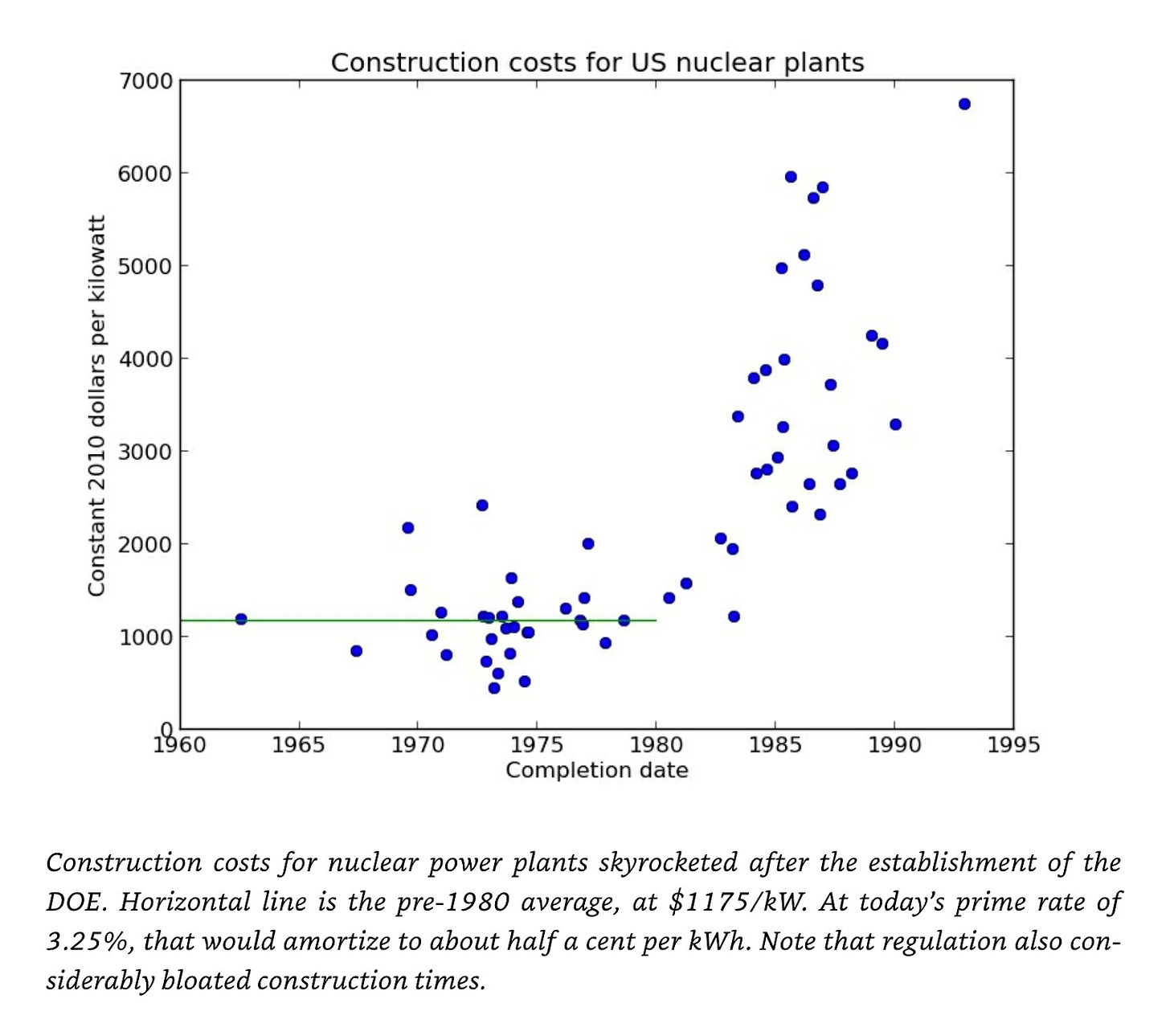
Tomas Pueyo: This question around correlation versus causation is interesting. Is it that human GDP can grow and it happens to be accompanied by growing energy consumption, or is it really the energy growth that enables GDP growth?
Jason Crawford: I think that the causation is actually reciprocal, and that these things feed back on each other and feed back on themselves in reinforcing cycles. And that's where progress can be exponential, and, over the longer period of history, it’s actually super-exponential.
So yes: energy technology makes possible other technologies, such as materials and manufacturing and construction. And then those technologies make possible more and better energy technology. Similarly, advances in science make possible energy technology and then energy technology can make advances in science and so forth. All of these things are in these intense feedback cycles.
Tomas Pueyo: Let’s imagine that, in five years, Helion—this company that Peter Thiel just invested in through his fund, Mithril Capital—figures out fusion and that energy costs divide by 100. What would happen?
Jason Crawford: I just did a little Twitter thread on this, where I was asking people for feedback on what we could do with energy abundance. What if we had at least 10x or more energy per capita available? There are a lot of really interesting answers, and some of the best are about powering industrial processes.

For instance, water desalination. If you have enough energy, you can take saltwater from the ocean and turn it into clean water, to pure water. We could have water everywhere for human consumption, for agriculture. We could use this to irrigate the desert. We could reclaim the Sahara.
One of the interesting decarbonisation efforts, from a company called Terraformation, is to plant an enormous number of trees, say a trillion trees, and to do so by reclaiming the desert through desalinated water. They are planning to do it with solar power, but you could just as easily use nuclear—either fission or fusion.
There are other interesting materials and elements in places like seawater or rock, or even in our garbage in landfills, and the reason we don't extract them is that it is not economical. And the reason for that is often energy costs.
If we had cheap energy, one of the interesting minerals that we could extract from the ocean is uranium. Nuclear energy could pay for itself by giving us the energy to extract its own fuel out of the oceans. There’s a lot of additional uranium in the rocks on the ocean floor. All of it could be extracted with cheap energy.
The other thing we could do with enormous amounts of cheap energy is we can do the same thing to the atmosphere: we could pull trace elements out of the atmosphere, including, of course, carbon dioxide. We could go beyond the goal of stopping climate change. We could actually have a goal of climate control. I think the right long-term goal for humanity is climate control and weather control. We should be able to decide what is the ideal composition of the atmosphere and just set it to that, as if we had a thermostat.
One of the ways we would do that is through pulling carbon out of the atmosphere. So the challenge of this is that of course, carbon, for all of its effect on the atmosphere, is only 400 or so parts per million of the atmosphere. It's a very trace element.
Anytime you're trying to pull something out of a substance in which it is extremely diluted, it just takes a lot of energy. With enough cheap energy, we could do it.
There are other things even more out there. Things like plasma gasification. I need to research it, but apparently you could just turn any waste into its constituent elements. I think Max Hodak said it on Twitter

Another fascinating one is artificial agriculture. Rather than doing agriculture outdoors in the dirt, under the sky, in the rain, suffering the weather and pests, just do it indoors: vertical farming, greenhouse farming, etc. There are different approaches to this, but they all rely on creating a completely artificial environment and, in particular, supplying artificial sunlight, which is why you need enormous amounts of energy. You can do it somewhat efficiently because you can give the plants just the wavelengths that are used by the chlorophyll for photosynthesis.
It takes a lot of energy, but if the energy inputs are cheap, you can have a lot less water input, pesticides, soil runoff from fertilizer, you use less land, etc.
Tomas Pueyo: I saw a thread like this one, and one of the interesting usages that I heard was transmutation. Imagine you can have all the gold or the platinum you want.
Jason Crawford: Yeah, that was an earlier thread from Andy Matushak

I don't actually know if we have the technology right now to do sort of arbitrary transmutation, even given enough energy, but in principle it should be doable.
Tomas Pueyo: One of the things that I keep thinking about is the future of how we organize ourselves. Obviously, energy has been a massive source of how we've organized ourselves in the 20th century. For example, the Middle East oil that has determined a lot of the fate of the world. What do you think might happen to our geopolitics if energy prices fall? For example, it’s going to be harder for Saudi Arabia or Russia to have the aggressive, expansive geopolitics that they have today. Can the past inform us on what's going to happen? How have these energy revolutions influenced our politics, and how do you see these can help us understand what's going to happen?
Jason Crawford: First off, yes, I would hope that a revolution in non-oil energy would hopefully decrease the political clout of Saudi Arabia in particular, and the Middle East in general.
If we were running the world on fission, fusion, or solar, for that matter, presumably you wouldn't be depending on these long pipelines that go from certain regions to others. Russia wouldn't be able to cut off Ukraine's energy supply, for instance, because energy could be much more local and decentralized. Fuel wouldn't be this rare commodity that only comes from certain regions of the world. I definitely think the Middle East has had an outsized influence on geopolitics over the last several decades.
But that's a relatively recent thing. If you look more broadly at how energy has influenced geopolitics, I think it's not just energy. It's technology generally: regions of the world that were on the technological frontier were able to dominate militarily.
One little island off the coast of Europe was able to dominate a lot of the world for a century or more because they had the wealth and the technology. One interesting question about major developments is: will they happen evenly? Who will get them? Who will be the first actor—whether it's a nation-state or whoever—and what will they do with that?
Tomas Pueyo: As we're talking here about these technologies, you're blurring the boundaries between energy and other technologies. That makes me think: what even is an energy technology? For example, was the invention of agriculture an energy technology?
Jason Crawford: I don't think of it that way. I think of technology broadly in buckets, like agriculture, manufacturing, energy, transportation, information, and so forth. In my mind, energy technologies are things like, in the pre-industrial world, wind, water, and muscle.
You could maybe argue that the horse collar was an energy technology, and that it let us harness draft horses better. Certainly, windmills and watermills were energy technologies, and maybe some of the mechanical gearing that helped us take that power and use it for things like a grain mill.
Besides that, there was not much in the way of energy technology until the industrial revolution. The first really revolutionary energy technology was the steam engine, and there's a reason why it is kind of the quintessential invention of the industrial revolution, why it's the symbolic beginning of the industrial revolution. Not because it was the one central source from which all of the industrial revolution flowed. It was not. But because it's just so important and central and symbolic of what that revolution was about.
Tomas Pueyo: So for you energy was wind, water, and muscle. And so any energy that would free up muscle would be considered an energy technology?
Jason Crawford: The real challenge with wind, water, and muscle is that they're very limited in the ability to be scaled up and concentrated. Not to mention they're intermittent. But the bigger problem I think is the quantity.
You cannot make the wind blow faster. You can't make the river run swifter or stronger. You can't breed a much stronger horse within certain pretty strict biological limits. So if you want to get more horsepower for a particular application, and the only way to do it is by literally adding more horses, that’s hard.
For example, in the late 1800s, agricultural mechanization was advancing to the point where someone wanted to combine a reaping machine that cuts down the grain with a threshing machine—traditionally stationary—that threshed the seed out of the husk.
This is known as a combine harvester, and it's a relatively large and heavy machine, so large and heavy that to pull one through the field required literally dozens of horses.
It was really just not practical at all, except in a few locations on very large farms in California.
And that's about the only place that the combine harvester was used until the invention of the gasoline tractor. What engines really did was allow us to scale up the amount of power that could be applied to any particular application and give us essentially a limitless supply of energy that could be brought to bear on any particular problem and concentrate it down.
A lot of the progress in engines was progress in the power to weight ratio that is making engines smaller and smaller for a given amount of power output—from the very first steam engine from Thomas Newcomen in 1712, to James Watson’s improvements in the 1760s, to high pressure steam engines that were applied to locomotives, to the internal combustion engine, all the way to the jet turbine.
You're just getting better and better power to weight ratios, which lets us do more and more, from stationary engines in the days of Newcomen, to large locomotives, down to automobiles and airplanes.
Tomas Pueyo: So what are these big innovations we have? You have a steam engine and you say, obviously it gets iterated on over the 19th century. We have the combustion engine. What do you think are these big energy revolutions of the last two centuries and what have they enabled?
Jason Crawford: The big ones have been steam, oil, and electricity. And the one that should have been, but was stunted, is nuclear.
What steam did was that it allowed us to get mechanical work out of fuel. Prior to the invention of the steam engine, these two types of energy were completely separate. We had plenty of fuel for heat and light in the form of wood and coal and candles and so forth.
And then we had mechanical energy from wind, water, and muscle. The engine connected these two things. It allowed us to use fuel for mechanical work, which was previously not possible. We could replace wind and water and horses and cows and people with fuel.
The second great revolution was oil, and more generally going from unrefined solid fuels to refined liquid fuel.
The challenge with wood and coal is that they're solid fuels, so they're inconvenient to handle. They have to be hauled around in physical containers, they have to be shoveled or hauled in buckets or bins. And then when you burn them, they're quite dirty. They give off smoke, which is quite noxious, and they leave behind ash, which must be disposed of. This is because they have not gone through a refining process to separate out the stuff that actually burns from impurities.
What was different about the oil industry as it got started was that we were not just using crude oil, which is the stuff you get out of the ground. Crude oil is a mixture of many different substances that work well for different purposes, such as burning for engines vs. lighting.
But by the time of the oil revolution, around the 1860s, we had relatively advanced chemistry, which gave us chemical processes that allowed us to refine oil into its constituent parts.
In particular, the first of these was called fractional distillation. In fractional distillation, you slowly heat up a liquid and you take advantage of the fact that different substances boil at different temperatures depending on their weight. So the shorter hydrocarbons—which are lighter molecules—will boil off first and you can collect them. And then you slowly raise the temperature and the next heaviest ones boil off and so forth. You can separate oil out into what are called different fractions. Each one is a distilled substance, and that's why they call it fractional distillation. So this allowed you to separate out oil into gases, liquids, and more solid or sludgy substances, and different ones of these were good for different purposes.
It turned out that one particular fraction known as kerosene made an excellent lighting oil, and there was a desperate need for lighting oil in the mid to late 1800s. That was what the oil industry actually was built on. That's what Rockefeller did originally, kerosene for lighting.
Later, especially as electricity took over from kerosene as the preferred lighting method, they pivoted into gasoline for the newly invented internal combustion engine.
The internal combustion engine was the next generation of engines that depended on these refined liquid fuels. Contrasting these engines: steam engines have a huge boiler full of water, and you just literally build a fire underneath it, like you might build a campfire or a cook fire. They can run on anything that burns. As long as you can build a fire, you can run a steam engine. Some steam engines on farms were run on fence posts and corn husks. It'll eat anything. Some machines that were conceived to go through fields were designed to run on straw. They would go through the fields reaping grain and eating the fuel they cut down as they moved forward.
The challenge with these engines is they're large and heavy, and in big part that is due to the enormous boiler, which contains a bunch of water because of the inherent inefficiency of using water as a medium to transmit the energy.
The internal combustion engine cuts out the middleman. There's no boiler, there's no water. You just ignite the fuel directly in the piston to push it. But to do that, you need a liquid fuel that you can spray into the cylinder. You would not be able to do this with coal or wood because you can't spray it in there.
And then when it burns, it needs to do so fairly cleanly so that you don't have ash to dispose of. Refined liquid fuels like kerosene and gasoline were excellent for this purpose.
And so that was the fundamental technology enabling the internal combustion engine, which had a much higher power to weight ratio.
Fun fact: one of the early locomotives circa 1829 called The Rocket and invented by George Stevenson had about the same horsepower as the Ford model T—about 20—but the model T only weighed about 1200 pounds, whereas the Rocket weighed some 9,000 pounds. Same energy, much smaller package.
And then the third big revolution was electricity. Electricity did a number of things for us. I would say the most important is that it was a way to transmit energy instantly and very cheaply across long distances with fixed infrastructure.
Fuel, of course, could be transported because it's a physical product and you can transport it in bins or canisters or tanks, and eventually in oleoducs. But electricity allows us to do that with cheaper, fixed infrastructure, pretty much zero marginal costs, instantly, and nearly anywhere.
Consider Niagara Falls. It's the largest waterfall in North America. A lot of energy goes over the falls. How can you capture that and harness it and use it?
Prior to electricity, the best thing you could do is build water mills and maybe power factories onsite. And indeed there were plans to do so around the end of the 1800s. But that would have been fairly limited. What they did instead, in 1895, is they opened one of the first hydroelectric power plants. With that, they could provide energy of all sorts to places that are miles away like Buffalo, New York, 26 miles away. There's no way to transmit mechanical power from a waterfall over a distance of 26 miles. You can’t stretch belts and chains for 26 miles. But you can run power lines.
The other amazing thing that electricity did was it gave us all these new applications of energy, especially the electric motor and the electric light, replacing combustion engines and the burning of fuel inside the home for lighting.
The great thing about this is that electricity tends to be cleaner, quieter, and overall safer than combustion technologies. Even when you had relatively good fuels to burn, like gas or kerosene, they still gave off some smell, they were still a fire hazard. Electricity was just better along all those axes.
Imagine a vacuum cleaner that ran on a gasoline engine. It's just not going to work. With all the smoke and exhaust, you wouldn’t end up clean in your own home.
The first powered washing machines were mostly run on electric motors. Electricity was able to bring power inside the home and the office. Not to mention revolutionizing factories as well with electric machines and machine tools and so forth, replacing the old way of using power.
The old way of distributing power was to have a large central engine that ran a central power shaft that would run along the ceiling. It would run along the length of the building. All the machine tools would be hooked up to that shaft by belts. It was a fairly inefficient system, among other things because you were constrained to organize the factory along the line of the central power shaft.
With electricity, you could completely reorganize the factory according to the flow of materials. You could optimize the flow of materials and tasks throughout a production process, rather than having to have everything in one big line along the central power shaft.
So those are the three big revolutions: steam, oil, and then almost simultaneously with oil, electricity, which really made the modern world of energy.
Tomas Pueyo: Super interesting. You’re talking about many aspects of energy that matter, such as how transportable it is, how clean it is… We've only talked about the cost with regards to the future of energy. Are there other dimensions of energy that you think we could be improving in the future?
Jason Crawford: Yes. The other one that really comes to mind is energy density. So energy density in various forms has been an important part of this entire story. I've mentioned the power to weight ratio of engines. That is one form of energy density. The energy density of fuel is another, measured in joules per kilogram, right? One of the benefits of oil and gas is that they are very dense fuels. Jet fuel for instance, is something like 50 times as dense as batteries. And this is the basic reason why we don't yet have electric planes—because the batteries would be too heavy and flight really needs a good power to weight ratio, because of course you're fighting gravity to keep your machine in the air. Every bit of weight that you add just increases the burden.
Energy density is really the fundamental reason why nuclear is such an exciting technology. You get absolutely insane energy densities. Nuclear fuel is something like a million times as dense in terms of energy per unit weight as any combustion fuel. It's just like a discontinuity in the progression of fuels.
It's at the point where refueling a machine or a plant is not even something that you do as a daily or weekly operation. It becomes more like annual maintenance. A nuclear power plant might get a delivery of fuel every 18 months or something, whereas a coal plant gets them, I don't know, every week or so. The sheer energy density of the future opens up amazing new possibilities. There are nuclear power plants on the drawing board that would be so amazingly efficient that they would not need to be refueled literally for decades. You can imagine machines that would have a lifetime of fuel built in. You can have nuclear cargo ships or freight trains that are refueled annually
Right now, nuclear technology generation needs large size machinery. But if you could imagine over some decades of experimentation and engineering iteration that we could shrink it down, that would be really amazing. Maybe your iPhone does not need to be charged ever, or it doesn’t have a charging apparatus at all. It just comes with its fuel baked in.
Isaac Asimov actually predicted this back in 1964, when he wrote a futurism essay predicting 50 technologies of 2014, 50 years ahead. One of the things he said was, of course the appliances of 2014 will have no electric cords because they'll be powered by long-life batteries running on radioisotopes.
Ford actually made a concept car in the 1950s called the Nucleon, a nuclear-powered car that would go for thousands or tens of thousands of miles without ever being refueled. That's the sort of amazing world we could live in if we had a much more advanced nuclear generation.
For the last part of our chat, Jason and I touched on governance and the future of progress. This week’s premium article will cover that section, along with my take on what Jason shared about energy, and especially his concern about how the reduction in energy consumption might have thwarted innovation. Subscribe to receive that article!
Tomas, Bill Gates has been a huge follower of research and books by Vaclav Smil. Since you are investigating energy issues, I feel that should not be missed in your coverage.
An excellent blog that covers this topic, written by a physicist, is Do The Math: https://dothemath.ucsd.edu/ That was where I first heard the topic of energy density and physical limits of energy consumption growing equally along with GDP growth. Highly recommended!