The 2023 UN Climate Change Conference (“COP28”) finished last month with many promises that obscure a key fact: We could easily stop climate change right now if we wanted. If we don't do it, it's just because we don't really want to.
Here's how we could do it, in 3 steps:
1. Stop Adding CO2
CO2 comes from burning fossil fuels, which is mostly used for electricity, heat, and transportation.
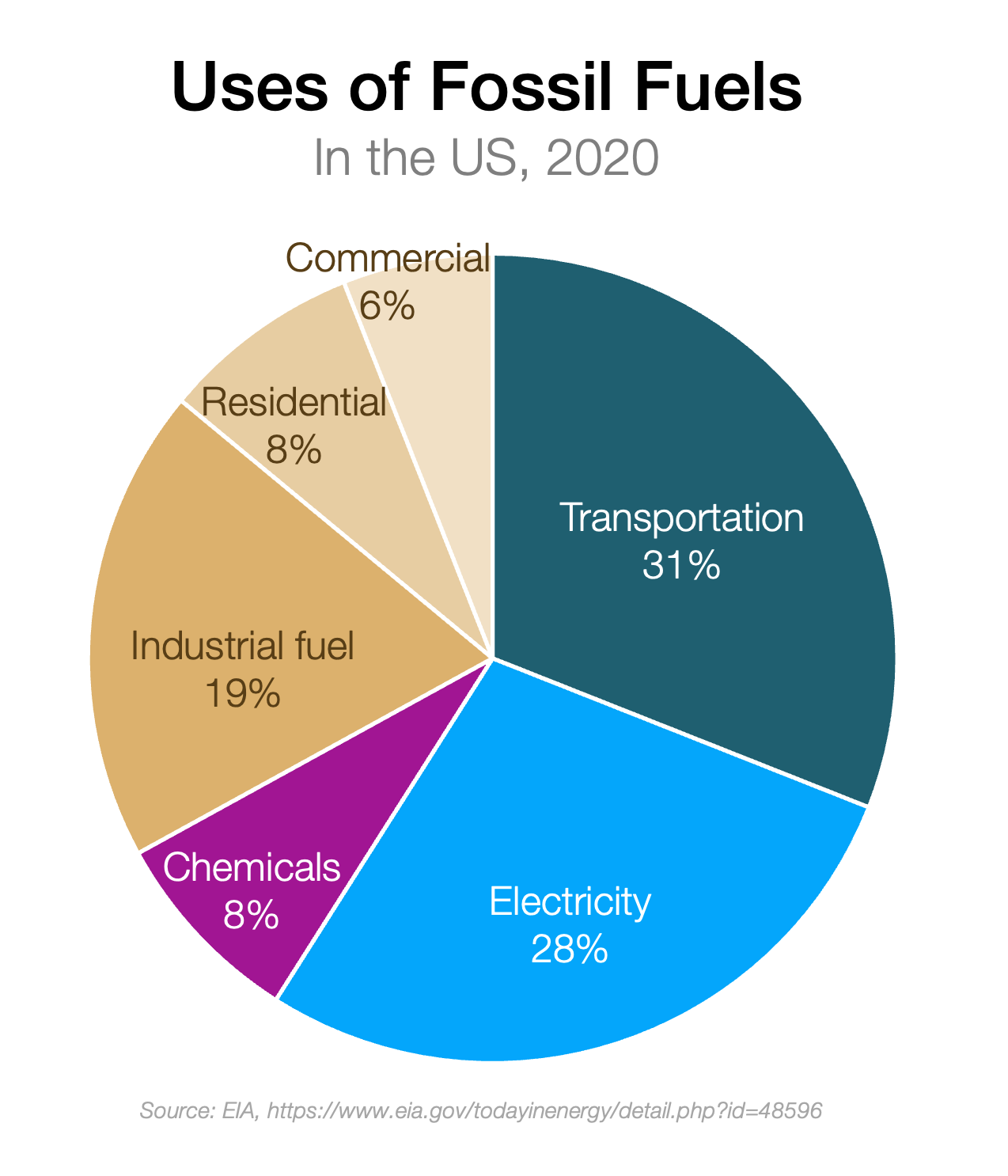
We need to stop the release of CO2 from each of these uses.
Electricity
It's a matter of time before all electricity comes from nuclear1 and renewables, especially solar.
Wind is also accelerating, but even combined they’re still far from making up most of the electricity generation we need.
If we want to accelerate this, we should add every clean energy we can, including nuclear2. Even then, it would take about two decades to mostly eliminate fossil fuels from our electricity generation.3
Heat
New technologies are appearing that use electricity instead of burning fossil fuels to generate heat, like heat pumps or high-temperature electrical heat, which are more efficient than burning the fuel directly.
It's a matter of time to deploy this tech at scale:
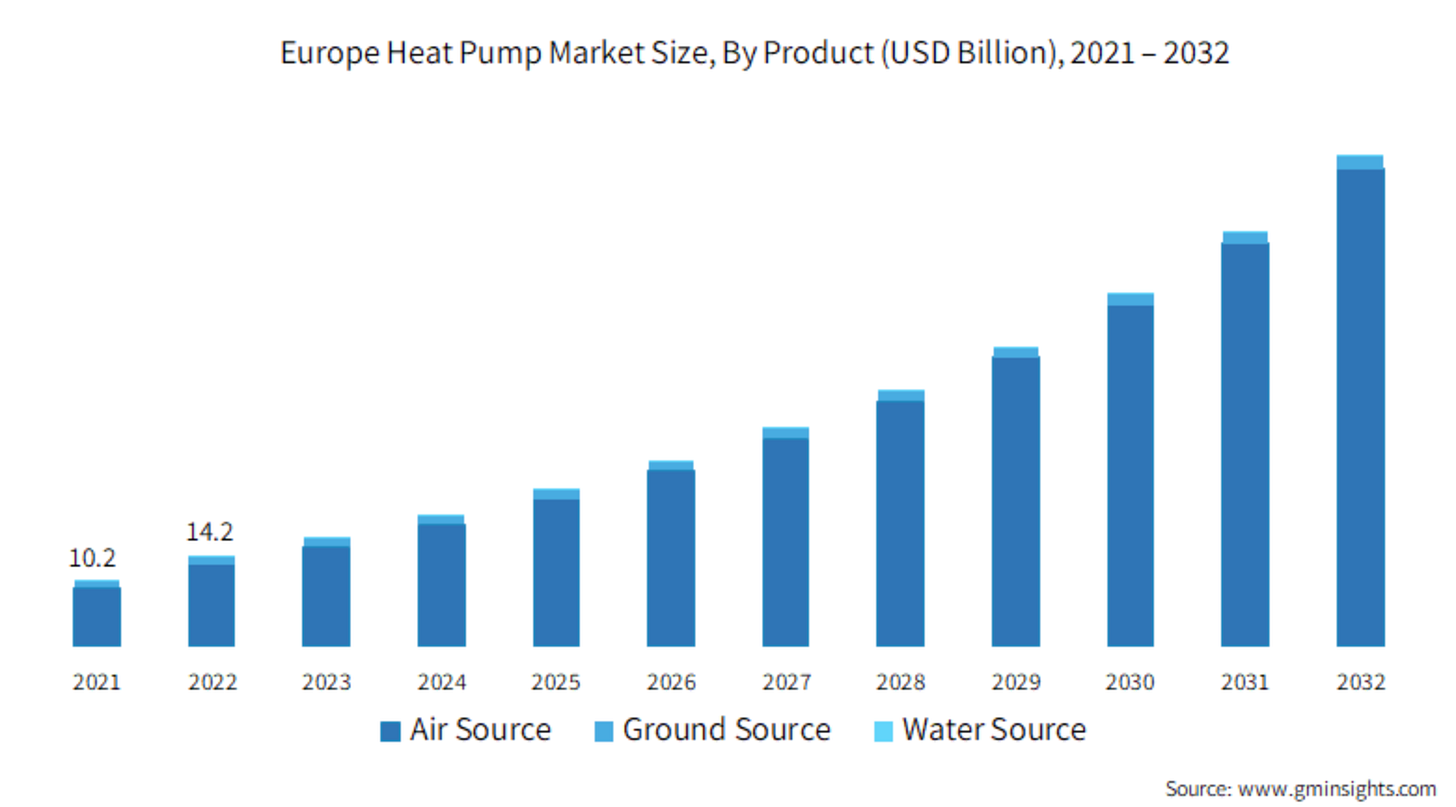
This graph is for the US. Europe is growing much faster.4
Heat pumps are great for residential and commercial heating, but when you need very high temperatures in a furnace to produce steel, they might not be ideal. As a result, the heat pump market for industrial applications won’t grow as fast as that for residential/commercial use.
But electricity can be used to reach high temperatures too, and electric arc or induction furnaces can be more efficient than fuel combustion ones.
There are other emerging sources of industrial heat. For example, designs for new nuclear power plants are exploring the possibility of reusing the throwaway heat in industry.
Eventually, industrial uses of fuel will be replaced by electricity.
Transportation
We’re already transitioning from fossil fuels to electricity thanks to the replacement of the combustion engine by electric cars.
The result is that a combination of renewable and nuclear energies, heat pumps, electric vehicles, batteries, and electric industrial uses can dramatically drop our usage of fossil fuels.
However, this transition will take time. Going back to the transportation example:
Electric vehicle sales are projected to pass internal combustion engines only in 2037.
And some transportation will never be electric. The batteries needed to fly a jumbo would be heavier than the plane itself. You must burn fuel to launch rockets to space.
So the transition away from fossil fuels will take time and be incomplete. Luckily, we don’t need to stop burning fuel.
Replacing Fossil Fuels with Synthetic Fuels
The problem with fossil fuels is not the “fuels” part. It’s the “fossil” part.
A lot of carbon was stored in the crust, and now we’re pumping it out and releasing it into the atmosphere. That’s the problem. But if we pull the carbon from the atmosphere, and then we release it back, that’s not a problem.
In Solar Energy Solves Global Warming, I explained how some companies like Terraform Industries can produce fuel from the air. The process is simple: You take CO2 from the air, you take H2 from water (you split it with solar panels), you mix the CO2 with the H2, and you get CH4, or methane, the main component of “natural gas” (except this would not be natural gas, but synthetic gas). You can then convert methane into other fuels like kerosene.
Since the fuel you’re burning came from the air (the CO2), not the ground, you’re not adding any CO2 to the air.5
OK, so within a couple of decades or so, we can dramatically slow down the addition of CO2 to the atmosphere with:
Heat pumps
Solar, wind, and nuclear energy
Batteries
New methods of industrial heat
Synthetic gas
And to get there, we don’t need to change the system! This doesn’t depend on any magical agreement between governments that never comes.
But we have still released a lot of CO2 into the atmosphere over the past two centuries!
The result has been a jump in atmospheric CO2 concentration.
It’s not enough to stop adding to it. We need to sequester about 2 trillion tons of CO2, or 2 Tt. Let’s remember that number.6 Transforming some synthetic fuels into plastics is not going to cut it. We need a faster way.
2. Sequester CO2
We need a way to sequester up to 2 Tt of CO2 fast and cheap.
Trees naturally capture CO2. Should we plant an awful lot of them?
If we converted 900 million hectares of land into forest, that would reduce the carbon in the atmosphere by 0.2 Tt. Good, but not enough. And it would take a long time for these trees to grow. And in fact, planting all these trees would not be enough, because the world is still deforesting. And 900 million hectares is already an awfully big surface, the size of China.
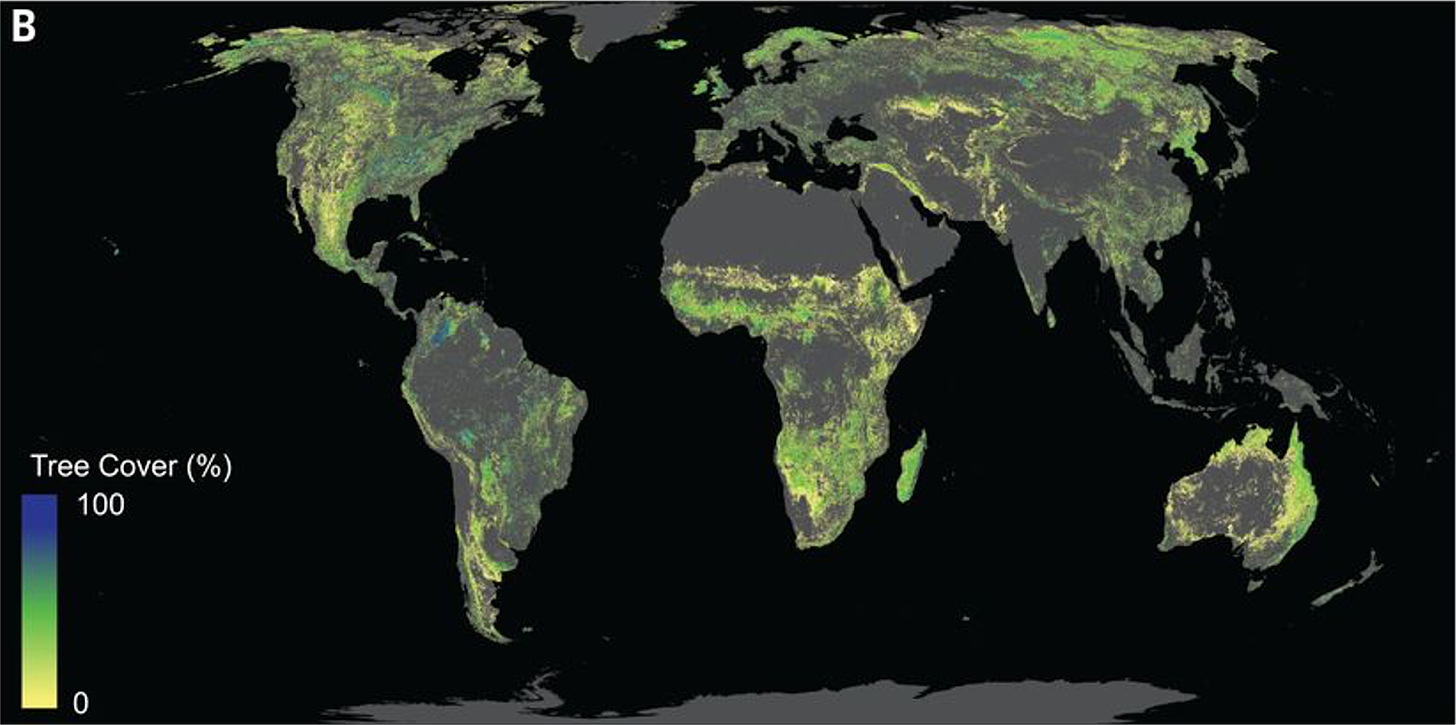
Not fast, not cheap.
How cheap does it need to be? Well, a ton of CO2 has a social cost7 of about $185, and the price of the CO2 ton in the market in Europe8 is about ~$30-$70. So we need to sequester CO2 for a much lower cost than these.
Current methods of carbon sequestration suck air, push it into a material that captures the CO2, and then consumes energy to release it and store it deep underground. That process costs hundreds of dollars despite being in places where energy is very cheap. Running this process at scale would be too energy-intensive: Our 2 Tt of CO2 would require 16 times the current annual energy production on Earth. Instead, you want a process that just grabs the CO2 and keeps it in a convenient place forever.
What about doing it the natural way? The way the Earth has been doing it for millions of years? Even to this day, the Earth naturally sequesters about 300 Mt (0.0003 Tt) of CO2 per year naturally. How? When the CO2 dissolves into rain, it naturally fixes to rocks.
Nerdy chemistry interlude
Jump this section if you’re not interested in the chemistry of how this would work.
First, the CO2 in the atmosphere mixes with water.
It forms a weak acid called carbonic acid.
When this hits a rock like olivine9, which looks like this:
…what happens is the carbonic acid steals the Mg atoms, and the Si atoms end up alone with some O, and become sand (SiO2):
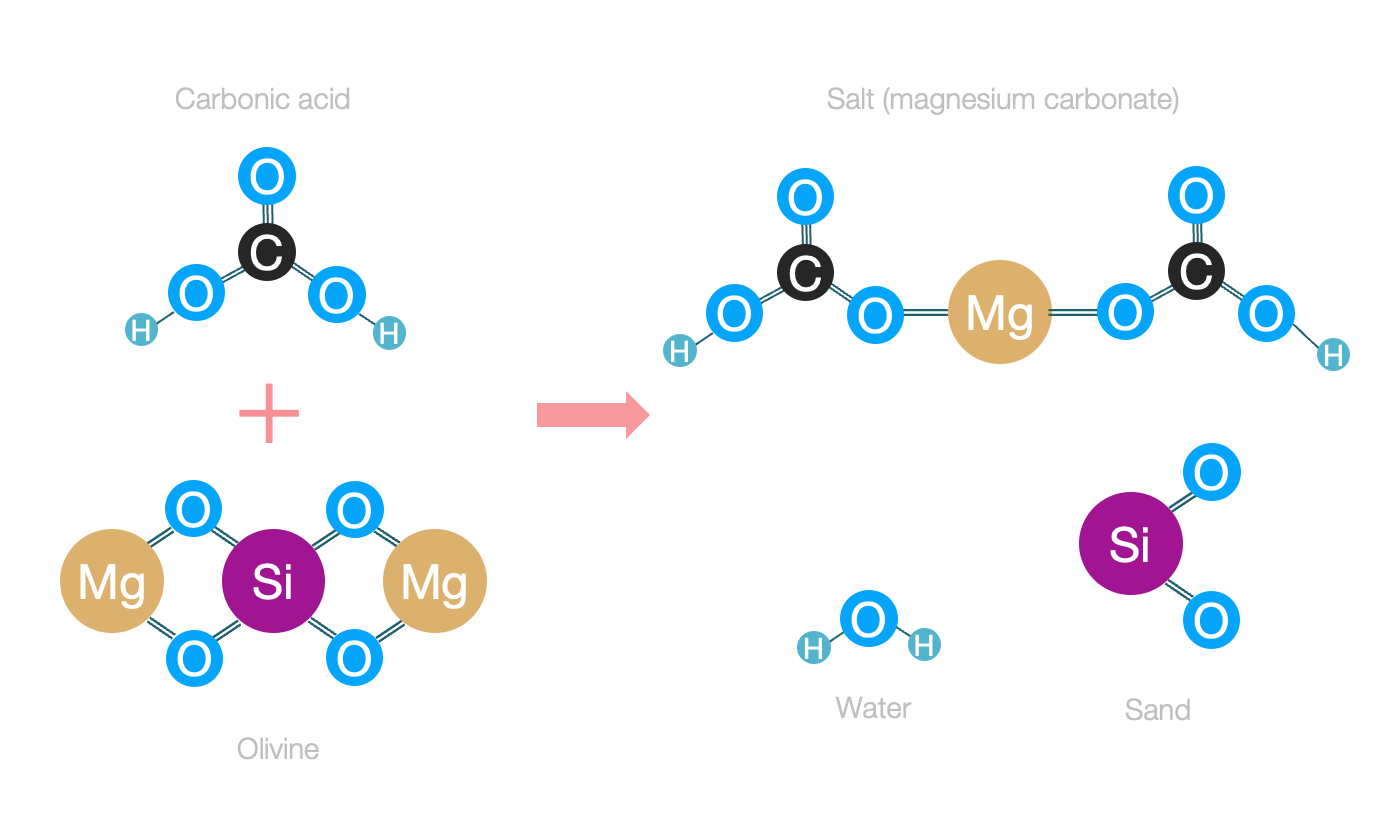
The result is magnesium carbonate Mg(HCO3)2 , which is a perfectly normal salt, with leftover water and sand.
End of nerdy chemistry interlude
One ton of olivine can sequester 1.25 tons of CO2, and olivine is everywhere. We just need to nudge nature a bit to sequester CO2 faster.
How? Chemical processes happen faster with heat, in liquid, and when there’s more surface area for action between the chemicals to occur.
We find hotter water in oceans close to the tropics, so that’s where we should drop our olivine. We should do it close to the coast to make transportation cheaper, and in shallow waters so that water remains warm where the olivine falls.
The only thing left to do is to grind the olivine small enough so as much as possible reacts with the CO2:
How small? 5-30 times smaller than a grain of sand.
How much olivine would we need to make this happen? About the size of a small mountain.10
How expensive would that be? About $9/ton of olivine, or about $7/ton of CO2. Which pales in comparison with the current cost of CO2, which is in the tens or hundreds of dollars per ton.
The total cost of the operation would be about $14 trillion as a one-off. Compare that with the current world GDP of about $100T; it’s doable, because it would be over several decades, or even centuries11.
The biggest source of this cost is energy. If we shrink that cost as explained above, this process would become trivial. At the cost of nuclear energy from the 1960s (adjusted for inflation), it would cost about the same as the wars in Iraq and Afghanistan combined.
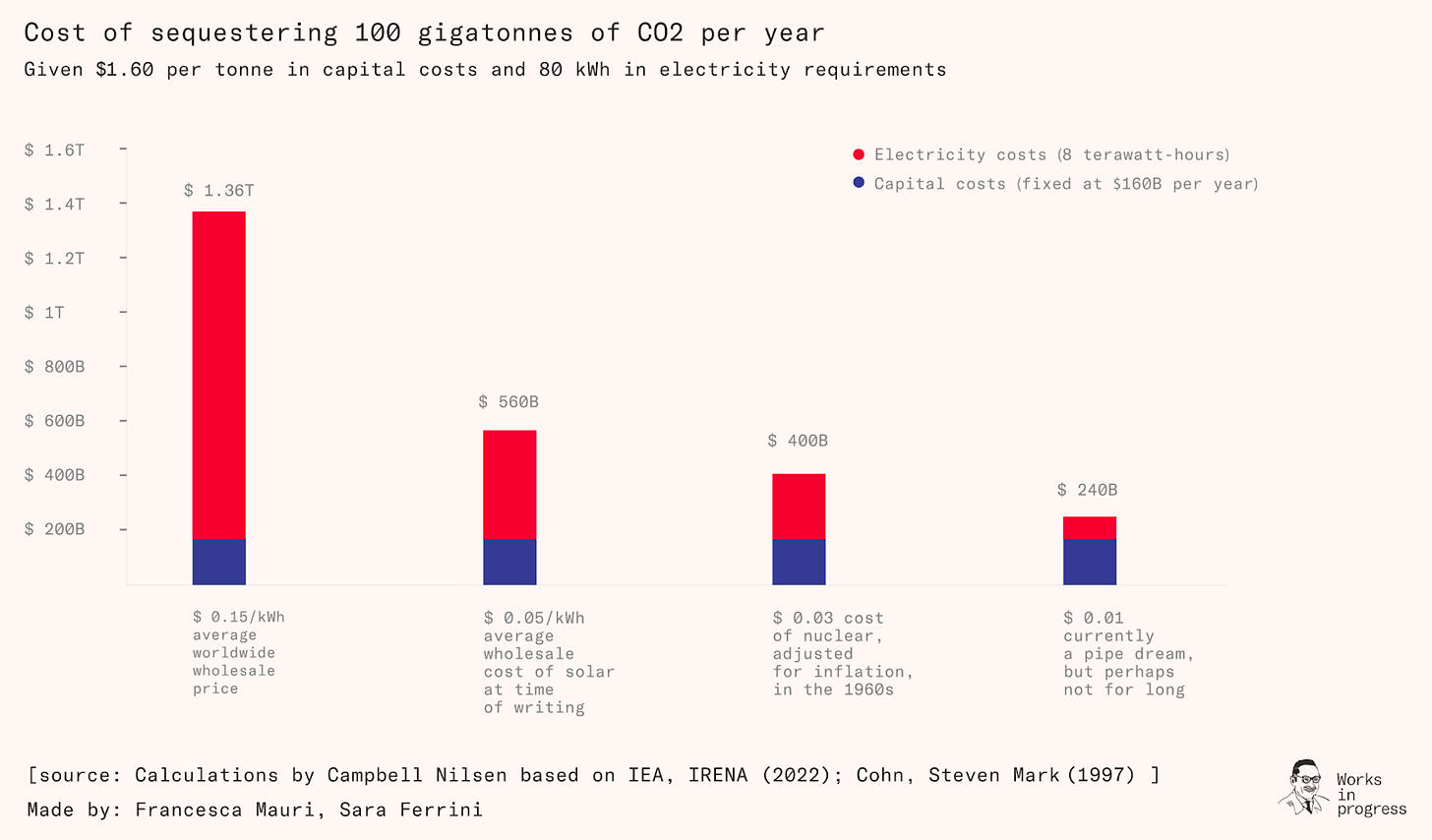
As you know, I’m a big fan of throwing soil in the ocean. The Sahara does it all the time, as do volcanoes, and that enables life. Throwing ground rocks into the sea can also enable life, which sequesters CO2. We could combine forces and try to throw soil to the sea for both chemical and biological CO2 sequestration: Chemical sequestration binds rock to CO2, and biological sequestration engenders life that sucks in CO2.12
But we could accelerate the process even further: There are many other ways to sequester CO2, like the reforestation we discussed and charcoal production. We could accelerate all these processes and have an even healthier Earth afterwards.
So we basically know how to stop adding CO2 to the atmosphere, and how to sequester what we’ve already released. It’s just going to take time—a few decades—and we don’t have it. So we need a stop-gap solution while we do all this. We need to cool off the Earth while we deal with the CO2, unless we’d rather gamble with what would happen if the ice caps and Greenland melted.13
3. Cool Off the Earth
Ramping up solar, wind, nuclear, heat pumps, electric cars, batteries, syngas production, and other such technologies will take time. We face at least 20 years of increased CO2 concentration in the atmosphere. There is nothing realistic we humans can do about it. So we have two options: Gamble with the Earth and the future of humanity, or develop a stop-gap solution.
We need a stop-gap solution: A way to reduce the temperature of the Earth while all these other technologies ramp up. The concept is simple:
The Sun heats the Earth14 and the Earth emits some of this heat back to space
The Earth warms up when the heat from the Sun is higher than what the Earth emits
To cool the Earth off, we can either let less sunshine in, or radiate more heat into space
Today, the Earth is warming because CO2 increases the amount of heat held by the atmosphere
We’re already trying to radiate more heat into space (by reducing CO2 emissions), and that will take time
Therefore, the only viable way to cool off the Earth in the short term is to reflect more sunshine
We don’t need to reflect that much sunshine. Just about 2%.
So how can we do it? A paper looked into all the possible ways to cool off the planet, and the two best ones were ways to reflect sunshine:
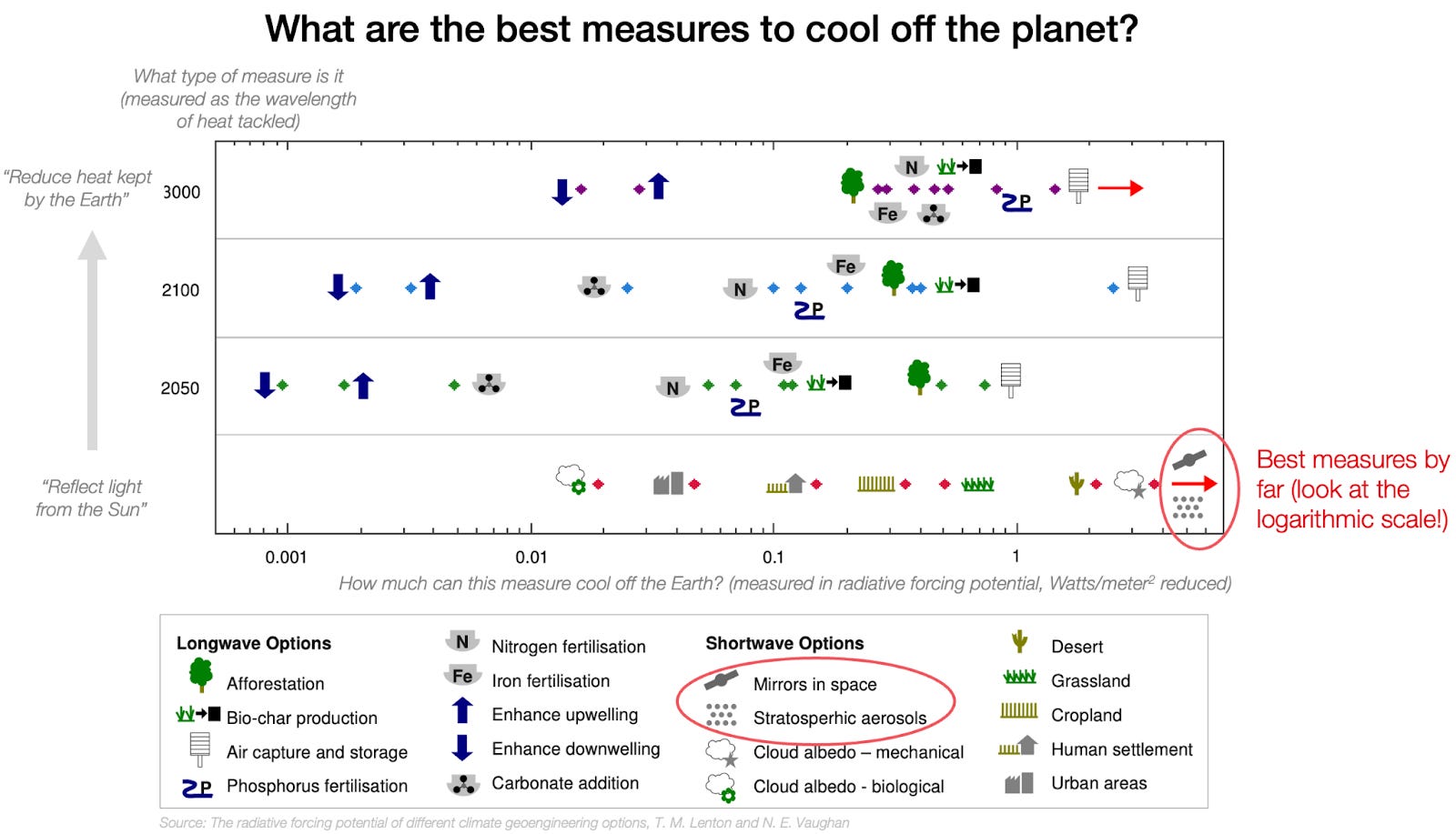
It concluded that mirrors in space and stratospheric aerosols are the best ways to achieve this by far.
Now I don’t want your head to explode, so let’s leave mirrors in space for another time. But just so you know, it would actually not be so hard, and we could do it in the next few years.15
This leaves stratospheric aerosols. Now we’re talking.
Did you know there’s already a natural way to quickly cool off the Earth?
Volcanoes naturally release a lot of stuff into the atmosphere, and that can reduce the temperature of the Earth. Remember how we said we just need to reduce sunlight by 2%? This is what volcanoes can do:
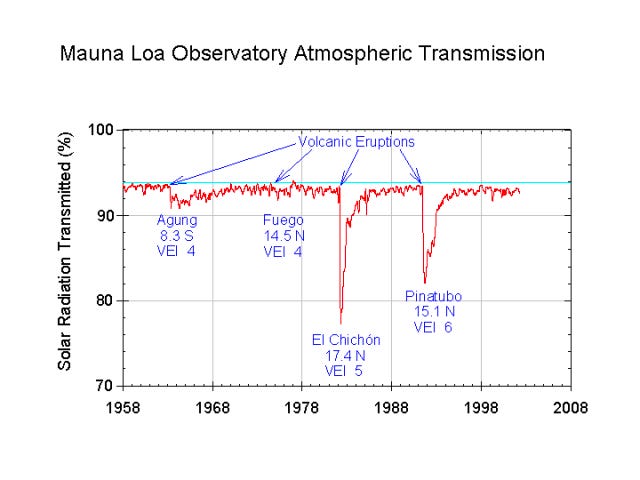
The main way they achieve that is by releasing SO2. The idea would be to do exactly the same thing as volcanoes do, except constantly, not with one-off explosions.
What’s beautiful about SO2 is that it reflects a lot of sunlight. Every gram you release in the stratosphere reduces the warming of one ton of CO2. A million times its weight! How much would it cost us to get a kilogram of that stuff to the stratosphere, so as to offset 1,000 tons of CO2? $0.35! Remember, we talked about the ton of CO2 costing between tens and hundreds of dollars. Now a thousand of these tons would cost a thousand times less to offset for a year!
If you want to offset all the CO2 we’ve ever released, that’s 2 Tt of CO2, for which we’d need 2 million tons of SO2. At a cost of $0.35/kg, that’s $700M.
We can stop global warming with $700M.
To put this number in perspective, countries just committed more than 100x that amount in the COP28. It’s 0.01% of US government spending! It’s nothing!
How would we do this? With balloons. We could have them tethered to ships with fans pushing the SO2 out, or simply fill balloons up with hydrogen and SO2 so they reach the stratosphere, and burn the hydrogen to release the SO2. That high, the SO2 would take a year or so to decay, which is great because it doesn’t accumulate. When falling, it wouldn’t cause acid rain like when SO2 is released in the lower atmosphere (where it quickly lumps and falls in a concentrated way). It would spread across the Earth’s stratosphere and dilute so that it would barely increase acid rain. We would release it close to the equator, because that’s where the most direct sunlight hits the Earth, and because air around the equator rises, so it would distribute the SO2 around the world.16 A company already offers you to offset your carbon footprint this way for quite cheap.
Conclusion
We need to solve climate change immediately, and we can do it in three steps:
Stop adding CO2 to the atmosphere
Sequester the CO2 we’ve already accumulated there
Cool down the Earth while the two previous points kick in
On the first point, we are on track to stop adding CO2 in the coming two decades through technology and capitalism, thanks to solar, wind, and nuclear energy, as well as other tech like heat pumps, batteries, electric cars, synthetic gas generation, and others.
On the second, we can sequester a lot of CO2 by accelerating a natural process, and simply grinding a literal mountain of olivine and throwing it into the sea. This will take several decades.
So we need an interim solution, which is to cool the Earth through aerosols—probably SO2— for just $700M (dirt cheap).
If you hear anybody complaining about how we can’t stop climate change, show them this.
If they start complaining about nuclear, capitalism, grinding mountains, or irrational fears of acid rain, tell them to study these solutions, or they’re just posing and don’t really care about climate change. If you want to talk with your local politicians about actual ways of solving climate change, share this.
This post was originally inspired by Casey Handmer’s We should not let the Earth overheat!
I have an upcoming article about nuclear.
Hydropower is mostly tapped out. Geothermal is too nascent.
Based on the current exponential path of wind and solar, and nuclear construction times seen in places that don’t penalize nuclear energy, like Japan or South Korea.
As we saw in Modern Energy Revolutions, it’s now cheaper in many places to burn gas to turn it into electricity, and then use this electricity in a heat pump to generate heat, than to burn gas in the home to extract its heat. This is how efficient heat pumps are, and it’s why they’re growing so fast.
You’re even extracting some of it, because some fuel becomes plastics, which are not burned into the atmosphere.
That’s like 2,000 billion tons (or 2,000 GigaTons, Gt), or 2,000,000 MegaTons (Mt).
It measures the monetized value of the damages to society caused by an incremental metric tonne of CO2 emissions and is a key metric informing climate policy.
There is a CO2 cap-and-trade market in Europe, where CO2 is priced.
I’m using the word olivine because most people use this. It’s a type of rock with Mg2SiO4 composition, but one or both of the Mg atoms can be replaced with Fe. Usually, people refer to the rock that only has Mg, and is called fosterite. But I find it confusing to call it sometimes olivine and sometimes fosterite, so I stick with olivine.
To sequester 2 Tt of CO2 requires 1.6 Tt of olivine. Let’s assume olivine weighs 2,700 kg/m3. That means we need about 600 M m3 of olivine. A mountain has broadly the shape of a cone. Let’s assume its slope has a 30º angle. Then its volume will be πH3, with H being the mountain’s height. So H= 3√(600,000,000/π)=573m. A mountain of olivine less than 600 m high (about 2,000 ft) would be enough. This is smaller than we’d imagine. Humans currently burn 8 Gt of coal every year. 1.6 Tt is about 200 years of present-day coal consumption in terms of mass.
These are, of course, back-of-the-envelope numbers.
I wonder if ferrous olivine (fayalite), Fe2SiO4, could achieve both. I’m not sure, because in both cases the iron atoms (Fe) are the active ones, be it when consumed by phytoplankton, or by carbonic acid. I also read that throwing fayalite into the ocean might be bad because of ocean acidification. I’m not sure. I think the principle here is that we can throw ground rocks at the ocean for both chemical and biological CO2 sequestration, that biological sequestration requires elements that are needed but rare in the sea like iron or maybe phosphorus or nitrogen, while magnesium is a strong candidate for the chemical type. If companies start doing this, one of the first priorities would be to find the precise rocks that optimize these processes.
This entire section is heavily influenced by Olivine weathering, an article from Works in Progress.
Actually the Earth also heats the Earth, as in the core is hot and some of that heat is pushed to the crust. But it’s not viable to reduce heat transfer to the crust. In fact, we might want to do the opposite and use more of that heat for our energy needs.
If such a reduction in incoming solar radiation were achieved by placing a sunshade consisting of multiple flyers at the L1 point (Angel, 2006), it would require a total area of 4.7 million km2, less than two Argentinas. It sounds scarier than it is. We could have very thin space sails sent to the Earth’s L1 Lagrange point. Sails ~30 m in diameter with a cell phone based guidance computer and integrated LCD panels for steering and trim would weigh 1 kg. Each Starship could launch 100,000 of these (soon after 150,000) with a combined area of almost 100 km^2 for each launch. So we would just need 15-20 Starship launches to put these mirrors in place. Idea from this article, again from Casey Handmer, who has thought about these topics harder than most.
SO2 also eliminates O3—ozone—but these concentrations wouldn’t have a big impact on the ozone layers at the poles. Also, the SO2 decays, and the O3 can regenerate. It’s also better to keep our Earth’s temperature than to maintain perfect ozone layers over melted ice caps. Worst case scenario, we can use alternatives to SO2 like calcite, but they have their own drawbacks, like cost and UV light absorption. The two best articles to read in depth about all of this are this one from Casey Handmer and this one from Nephew Jonathan. This paper is also a good summary of all the potential geoengineering solutions to climate change.
Interesting read. A UN-approved report last year said that geoengineering by injecting sulphur dioxide into the atmosphere may be necessary, but would come with major uncertainties. Releasing S02 is not without risks which we don't fully understand, and some regions might 'lose' in terms of greater climate impacts as a result. 'The primary challenges of geoengineering are conducting field experiments to accurately assess potential consequences and developing international agreements to safely deploy and monitor geoengineering technologies.'
https://www.nature.com/articles/s41558-019-0398-8
https://www.theguardian.com/environment/2019/mar/11/solar-geoengineering-climate-change-new-study
https://sitn.hms.harvard.edu/flash/2022/reversing-climate-change-with-geoengineering/
https://www.ucl.ac.uk/news/2020/mar/right-dose-geoengineering-could-reduce-climate-change-risks
Also, as mentioned by others, climate change is only one of the many planetary boundaries we are exceeding; others include novel entities, biosphere integrity, land-system and freshwater change, and biochemical flows (phosphorus and nitrogen). The debts are mounting up as our environment (water, land, air, oceans) degrade and can support less. Surely we need a more fundamental systems change from our current economic growth model at all cost to something more sustainable.
https://www.resilience.org/stories/2023-09-18/the-earth-system-has-passed-six-of-nine-planetary-boundaries/
I'm amazed that conservation is rarely mentioned in much of what I read. It must be more efficient in many cases, at least up to a point. Do we ignore it because it is just 'too hard' in our current economic system?
Thanks a million for writing this. I am retired, but up to late in 2021, I worked over 20 years in patent portfolio management for a Fortune 500 company, after having been trained as a research scientist and practiced as one (for that same company) for 15 years. One of my internal client groups, as a patent portfolio manager, was the huge, sprawling Engineering team - the folks who did the very serious work of designing, building, maintaining, troubleshooting, and improving all the "must work" hardware that goes into factories that are actually making stuff for the company to sell to people and other companies. The folks I had regular contact with were SERIOUS engineers. No "pie in the sky" for these folks. They worked on things that needed to be worked on in order for the company to make money in the short, medium, and long terms, and the things they toiled on had to work. Not just theoretically. When someone threw a switch, stuff had to work.
I was always taken, when "chewing the fat" with these folks, by how many of them dismissed as "solutions" most every thing in your "Part 1" that is commonly put forward as being responsive to the threat of climate change - ESPECIALLY the things put forward most avidly by experts in environmental sciences. The common complaint was that they were good ideas, but "drops in the bucket". The most common explanation for why they were always the things that "experts" (with educations equal to their own) were putting forward, was that the experts are "too close to the problem" and "dependent for employment on companies and agencies tied to those approaches". Almost to a person, these engineers believed that - eventually - the world will do your "Part 3", but likely, not until the pain of climate change is very, very great. Because... politics...
We need more folks like you making The Big Picture clear - or at least clearer. Just because something is good to do, does not make it a solution to a problem. We need to approach this from a standpoint of "If the plan NEEDS to WORK (and it does...), what does the plan NEED to include?" My experience is that many, many well-educated engineers already "get" what you're saying here - and we need to get it better communicated to the rest of the culture.