This article will convince you of that nuclear is the best source of energy.1 Don’t read it if you need your mind to remain anti-nuclear. If you are against nuclear, I recommend you to write down your precise concerns and what it would take for you to be convinced that nuclear is great, otherwise you might move the goalposts subconsciously.
To do justice to the topic, the article had to cover all the important aspects of nuclear energy, and as a result is long. I chose to publish it in one piece despite that, so all the relevant information is in one place, and I can update it over time and you can bookmark, reference, and share it.
The next couple of weeks will be focused on exploring this article in particular and nuclear energy in general. I will do an Ask Me Anything session for premium subscribers next Tuesday, January 16th at 6pm Europe time / 12pm EST / 9am PST. More details to follow.
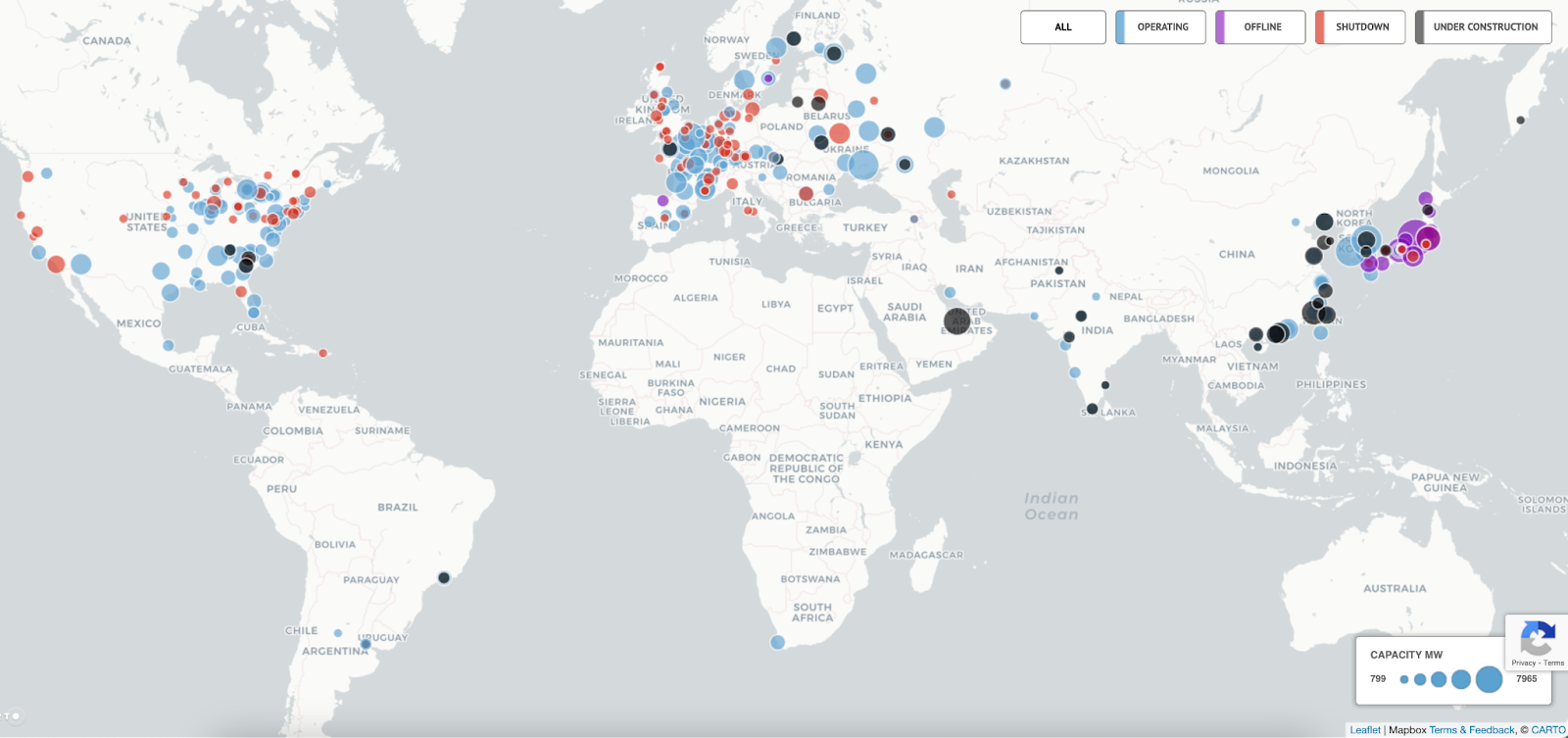
Nuclear energy2 is having a renaissance around the world: New reactors are under construction or in advanced planning in over 20 countries, including key ones like China, Japan, India, the UK, France, Russia, the US... At the UN's COP28 climate change conference, many of these countries signed up to triple global nuclear energy capacity by 2050.3
Why? Because people support nuclear energy in most countries:
And that support is growing:
Why so much support?
Nuclear is the best type of energy across every factor that matters: safety, environment, sustainability, reliability, politics, and economics.
Then why is it so polemic? Because understanding these aspects requires so much detail that they appear complex. In this article, you will learn all the details of why nuclear energy is the best.4 It boils down to this:
1. Safety & Health
Nuclear seems unsafe and risky because accidents appear monumental and the danger is invisible. It awakens a primeval fear in humans, like plane crashes. But when you measure actual safety, it turns out nuclear energy is among the safest sources of electricity.
Lowest Deaths per TWh
The best way to measure safety is by deaths per unit of energy (eg, deaths/TWh). What we get is energy, what it costs us is lives. We want to sacrifice as few lives as possible for our energy.5
Nuclear causes about the same number of deaths as solar or wind, about 100x fewer than gas, and 1,000x fewer than coal! Even something that appears as safe as hydropower is 40x deadlier than nuclear.
How is this possible, you might ask?
Let’s take coal, for example. It has killed about half a million people in the US since 2000—one death for every 3,000 households’ energy every year. How do we know? There are many deaths from mining, but they’re not the lion’s share. Researchers looked at the deaths of people downwind of coal plants, and noticed they had a much higher mortality, likely due to fine particles emitted by coal plants. They are now cleaner, but not perfectly clean, and people keep dying from their pollution.
When a train carrying oil derailed in Canada, it killed 47 people and destroyed half of a city’s downtown.
What about hydropower? What could be safer than just storing water behind a dam?
During Typhoon Nina in 1975, the Banqiao Dam broke, and after it 61 other dams downstream, killing between 26k and 240k people. Accidents keep happening.6
These two maintenance workers, aged 19 and 21, died installing wind turbines.7
Every energy source kills. Every job kills.
How is it possible that nuclear energy kills so few people then? What about accidents like Three Mile Island, Fukushima, or Chernobyl? How can nuclear energy be one of the safest, even when accounting for these deaths?
There have been thousands of accidents in nuclear plants. They happen constantly. Like with every piece of machinery. Of those, about 100 have been serious. Of those, about 10 caused deaths—in most cases, they were operators. Only three had non-worker deaths, two of them in the Soviet Union, and none after Chernobyl, nearly 40 years ago. Surprising? Let’s talk about Chernobyl and the other accident you might have in mind—Fukushima.
Chernobyl
Chernobyl is the 64th worst energy accident in history.8 In other words, 63 other accidents in the world of energy have killed more people than Chernobyl.
How many people died? Two plant workers died during the actual explosion of the nuclear reactor.9 Of all the emergency personnel who worked to stabilize the situation, 134 suffered from acute radiation syndrome (ARS). Of those, 28 died in the weeks that followed, for a total of 30. Within 20 years, 19 more would die, but most of them were likely not due to radiation: Some died of tuberculosis, others of stroke, heart attacks, accidents, and yes, five cancers. It’s likely that the majority of these did not die because of the Chernobyl accident.
Think about that. Only 21% of those suffering a massive amount of radiation died directly from it, and at most 35% died if you include all deaths within 20 years. 65% survived even very high levels of radiation!
Radiation might have increased cases of thyroid cancer in children by about 30%. But 92-98% of such thyroid cancer patients survive, so the number of thyroid cancer deaths in children might increase by a very small amount—about 100-385 deaths. These children would have been spared if they had been evacuated faster or taken iodine. To date, only 15 of these 100-385 deaths have actually happened.
What about the general population? No additional deaths, according to the UN. How do they know? Cancer and deaths from cancer did not increase closer to Chernobyl vs farther away. No effect on fertility, malformations, infant mortality, no increase in any cancer besides thyroid. This makes sense based on what we know today: Low doses of radiation are actually not a big deal, and might even be beneficial. It takes a big radiation hit to suffer.
So in total, between 30 and ~400 people died in Chernobyl. At most 49 were directly caused by the accident, and the rest was due to mismanagement. The worst accident in 70 years of nuclear energy history is comparable to a plane crash. And Chernobyl was unique because its design was a joke: It was unstable by design, because the Soviets were poor and skimped on all costs, including safety.10 Meanwhile, the operators were poorly managed, and the political system that controlled the plant was even worse.11
By the way, did you know eight reactors like Chernobyl’s (RBMKs) are still operating today? Operators figured out their mistakes, corrected them, and have used their safe energy for 40 years since.
What about Fukushima?
Fukushima
There might have been one death from the accident—a worker who died of cancer years after the cleanup. The accident might or might not have caused it.12 This is the extent of deaths after a historically huge earthquake and following tsunami hit the nuclear plant!
About 51 people died during the immediate evacuation of Fukushima. They died from things like hypothermia and dehydration. If most of them had stayed in their hospitals and care homes, they would have survived, because the level of radiation was so low that it was mostly harmless.
A total of 160,000 people were evacuated due to the earthquake, tsunami, and nuclear core meltdown, and 15,000 died. Of those, 2,300 died in the Fukushima prefecture, and ~1,400 of them received government subsidies linked to the nuclear power plant over the following years. Most of these deaths are from people who lost their home, their business, their social circle, prevented from returning by the authorities, and include things like suicide and other deaths of despair. All these deaths were preventable, since the levels of radiation surrounding the Fukushima nuclear plant are mostly safe, as we’ll see later.
What’s remarkable is that nobody died from the accident itself. As we said, one person might have died from it afterwards. The vast majority of deaths after the accident were because of the evacuation. It makes sense to evacuate so many people so fast after the destruction brought by an earthquake and tsunami, but in the case of the nuclear plant accident, it was an undeserved panic. Given that, I think the right number of deaths to allocate to Fukushima is precisely one. The number of Deaths/TWh I showed above includes 2,300 deaths from Fukushima, making it ultraconservative.
So in the case of both Chernobyl and Fukushima, the deaths were less caused by the accidents themselves, and more by the hysteria of the political management that ensued. Most nuclear deaths are probably not due to the overstudied reactor accidents, but by the forgotten day-in-day-out construction-related accidents.
But how risky are spectacular accidents like Chernobyl?
The Risk of Severe Accidents Is Extremely Low and Tolerable
Risk depends on probability and severity. The more likely and bad something is, the more you want to avoid it.
Nuclear accidents are both unlikely and not very severe.
Likelihood of Nuclear Accident
Accidents in nuclear power plants are a bit like those in planes. Most planes fly for millions of km without an accident. Every day, millions of people take to the air with the near certitude that they will land in one piece.
We know planes are safer than cars, but their accidents look horrific, and we have such a lack of control that we develop an irrational fear of them. That doesn’t change the fact: They’re extremely unlikely.
So far, we’ve had about 15,000 years of nuclear reactor operation, and about 10 core accidents, giving nearly one accident every 1,500 years of operation. This is mostly with old designs. New Generation 3 designs are over 50x safer—one in 100,000 years of operation. Generation 4 aims for an even lower probability, from one accident in a million to one in a billion years of operation.
How? Because of two reasons.
First, because we’ve been improving this technology for seven decades. We’ve learned a lot about what works and what doesn’t, and tirelessly eliminated every failure proven by experience. This is the same reason as why planes fell from the sky like flies in the 1970s, but they barely happen anymore.
Unfortunately for planes, if they stop working, they fall and everybody dies.13 This is not true of nuclear energy. The second reason why it’s safer now is because of passive safety measures. Now reactors are designed to shut down if anything goes wrong: No need for humans, electricity, machinery… These safety measures are based on reliable things like gravity. For example, if you put the reactor under a water pool, an explosion would drown it and cool it off.
So now we have much better nuclear designs, and we will have fewer bad accidents in the future. But even if an accident like Fukushima happened every 10 or 50 years, it would be OK, because nuclear accidents are not very severe.
Nuclear Accidents Are Not Very Severe
You should already suspect that the severity of a nuclear accident is low when the two biggest accidents, Chernobyl and Fukushima, directly killed 49 people and maybe 1 person respectively.
Once you understand14 how nuclear reactors work, you realize at a deeper level how safe they are.
A nuclear power plant cannot explode like a nuclear bomb. For that, you need something like near pure uranium U-23515, whereas power plants only use about 3-5% U-235. This is not enough for a runaway chain reaction to be triggered.16 If the nuclear fuel is left alone, nothing happens to it. You need to actively intervene for a chain reaction to be sustained.17
But if you make a mistake like in Chernobyl, you can accelerate the reaction. The worst that can happen in these circumstances is a meltdown: The uranium heats up and melts. This is problematic because it can overheat very quickly, which then boils the water used to cool it, which becomes high-pressure steam and explodes, carrying some radioactive material into the air. This is what happened in every famous core meltdown.18
And as we saw before, even the radioactive material that reached the atmosphere in Chernobyl didn’t kill many people, because humans can bear a fair amount of radioactivity.
So core nuclear reactor accidents are very unlikely, are becoming even less probable, and are not very bad when they happen.
You might wonder: Wait a minute, the problem with Chernobyl is not just the deaths. What about the exclusion zone? And what about radiation outside of accidents?
Radiation Is so Low It Doesn’t Matter
Did you know that the Chernobyl plant kept operating until 2000, 14 years after the accident?19 The workers protested their closure. Do you think they were scared of radiation?
Did you know that coal power plants are 10-100x more radioactive than nuclear power plants?20
That doesn’t mean coal plants are dangerously radioactive. The radioactivity of both is minimal.
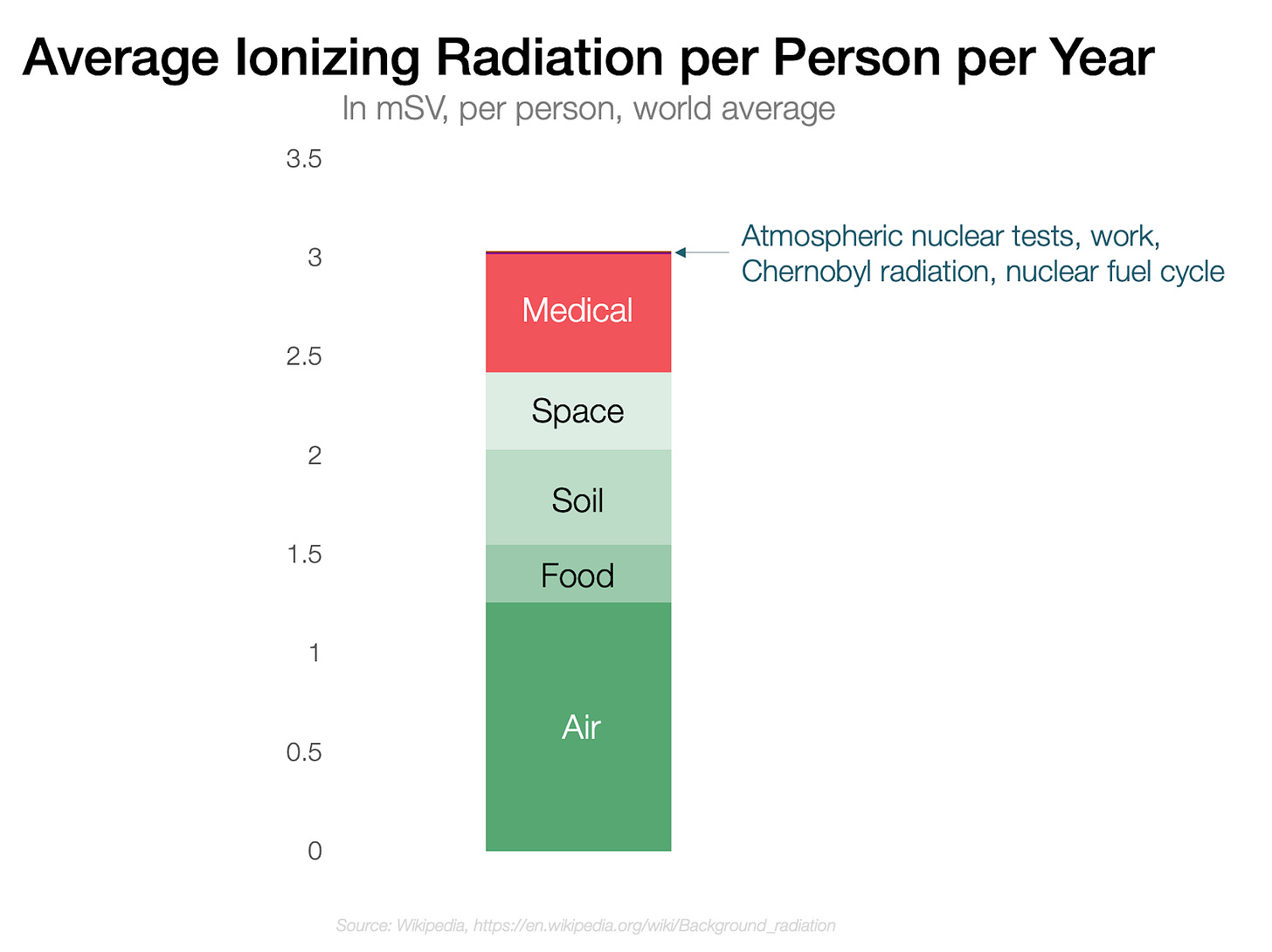
The average person receives about 3 mSv of radiation annually, of which over 80% is natural. That’s our baseline: 3 mSv/y. Remember this number and unit. I will use this reference and unit throughout the article.
Nuclear workers are usually permitted about 10x that radiation every year: 20-50 mSv/y.21 A whole-body CT scan will add 10 mSv/y, or 3x the average annual dose from nature.
In some places, there’s so much naturally occurring radiation that people are exposed to much more. In Ramsar, Iran, some people are exposed to 260 mSv/y! That’s 100x more than the average person! Do people in Ramsar have more cancer than others? No. In fact, a study showed they had half the level of genetic aberrations as those living nearby, in areas with less background radiation. The lowest level of cancer was in the highest radiation area. It might be that some exposure to radioactivity is good for you, as your body gets used to the attacks and gets better at correcting your DNA.22
In comparison, living within 50 miles of a coal plant gets you 0.0003 mSv/y; a fraction of that close to a nuclear plant. You get more radiation from eating a banana than living close to a nuclear power plant. In other words, radiation from nuclear power is completely irrelevant.
This graph has a linear axis. You can’t even see the effect of standard nuclear radiation.23 Here is a logarithmic graph, including Chernobyl radiation of first responders for comparison:
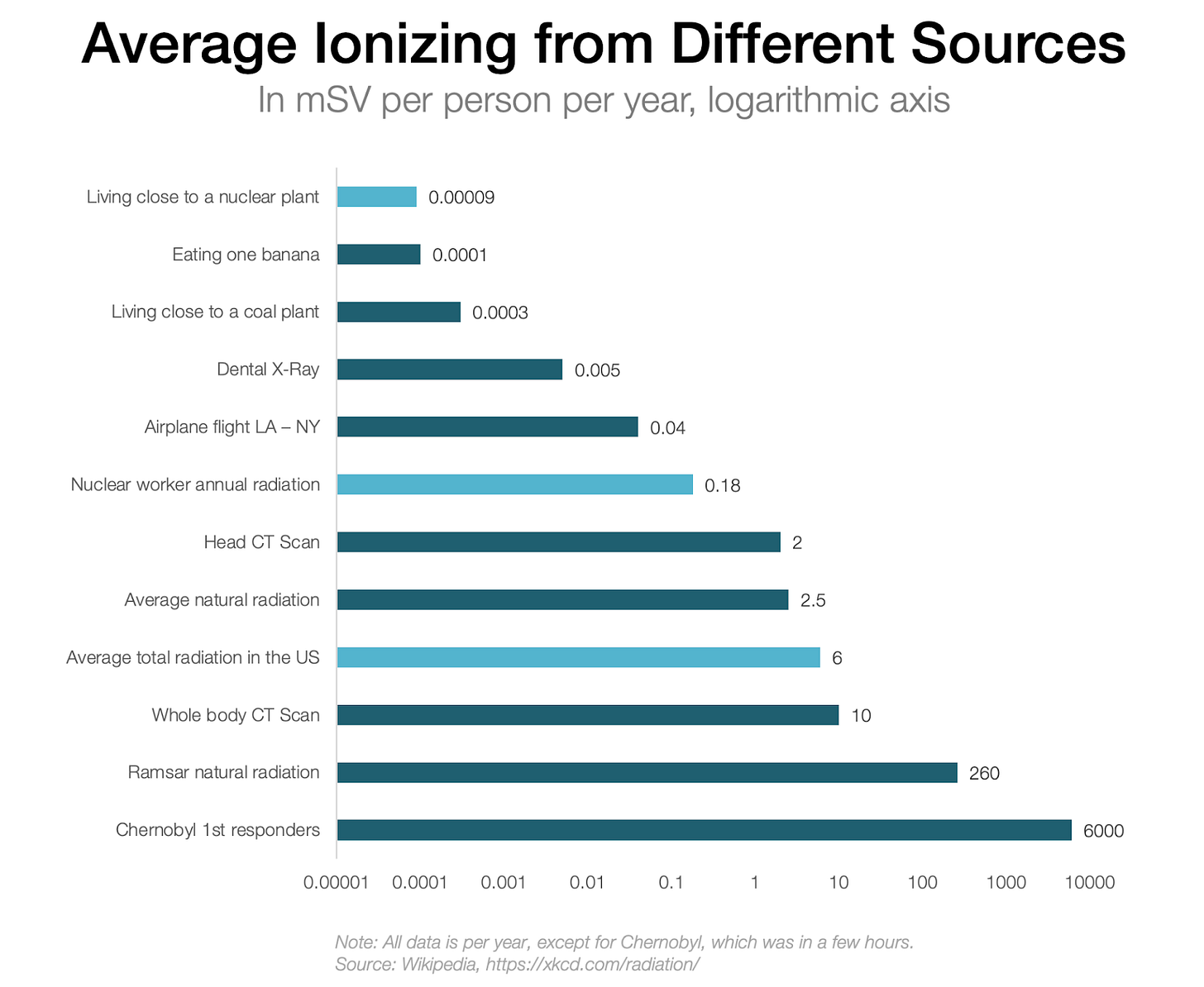
Why did Chernobyl first responders die and Ramsar inhabitants don’t? For two reasons. One is that Ramsar residents receive an order of magnitude less radiation. But also, it’s because the Chernobyl first responders got all this radiation within a few hours. It appears that the body can better cope with a bit of radiation, but gets overwhelmed if it gets a burst.
Some radiation spread out over time is OK. But concentrate that radiation into a few hours and you die. Another example is Hiroshima and Nagasaki. Of those who survived, the most exposed (within a 1 km radius of the explosion) got over 10,000 mSv in a few hours and 1% of them developed leukemia (only!). Those who were 2-3 km away got less leukemia than controls farther away!
These examples suggest that the impact of nuclear radiation on health is either non-linear (that low levels don’t harm at all and might even be beneficial), or at worst that the low levels of normal radiation we’re talking about are barely harmful.
If the people who live in Ramsar get one million times more radiation than those living within 50 miles of a nuclear power plant, nuclear power plant radiation is irrelevant.24
OK, normal nuclear radiation is so low it doesn’t matter. But before we were talking about accidents. Maybe radioactivity close to Chernobyl or Fukushima does matter?
Chernobyl
This is what the area looks like today:
Ah, no, sorry, these are the Przewalski's horses inside the Chernobyl Exclusion Zone. I meant to paste this:
All these animals are now thriving in the area: gray wolf, badger, wild boar, roe deer, white-tailed eagle, black stork, western marsh harrier, short-eared owl, red deer, moose, great egret, whooper swan, least weasel, common kestrel, beaver… Even the lynx and European bison. This is now Europe’s 3rd largest nature reserve. It appears that the presence of humans is a much bigger nuisance to them than radioactivity.
Chernobyl was a net positive for wildlife. And for tourism:
There is a big industry of people who visit the site of the explosion. Do you think the tourist guides are scared of the radiation they’re getting?
Not all the areas in Chernobyl have been so lucky. This is the Red Forest, in the immediate vicinity of the Chernobyl plant:
Twenty years after the accident, in the worst parts of the Red Forest, you could receive 10 mSv per hour. That’s about the same as living in a CT scan machine.25 Not great.
This map shows what radioactivity looks like today:
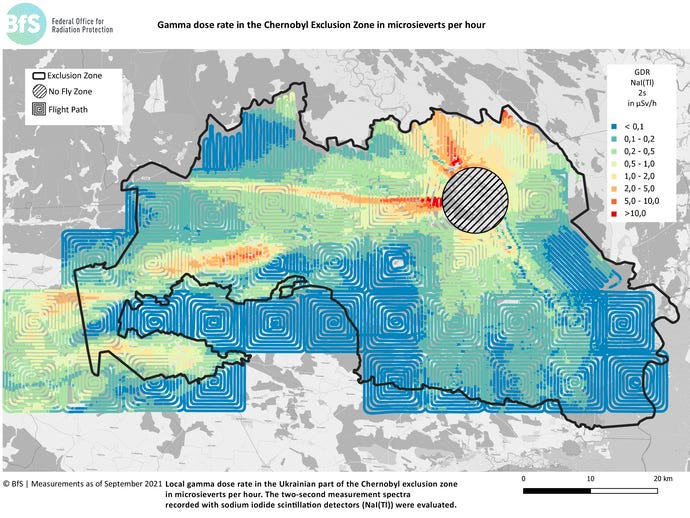
Here’s a satellite image of the area.
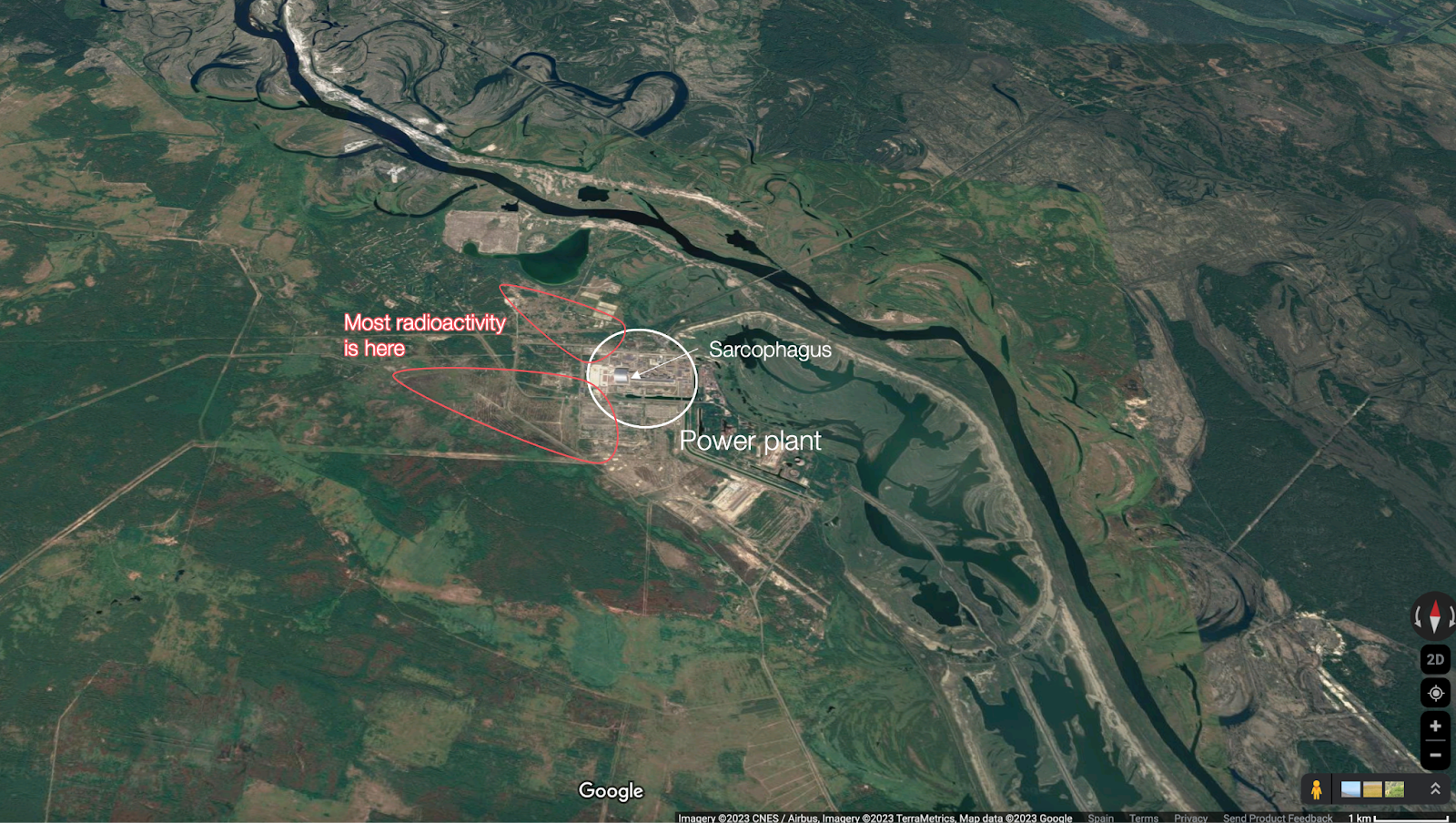
You can tell where radioactivity was highest because it is still reddish now on the satellite. This is really the only part of the entire area that might not be habitable today, about 5 km2. In other words, the worst nuclear accident in all of history, which is unlikely to ever reoccur, rendered about 5 km2 uninhabitable today, and this area will shrink every generation. This is not a lot, much less than what other sources of energy waste.
Chernobyl radiation is so low it’s mostly less than is naturally occurring in Ramsar, Iran.
Chernobyl radiation is so low it kept operating for years.
Chernobyl radiation is so low the area’s nature—and tourist guides—thrive.
Chernobyl radiation is so low Ukraine gets 50% of its energy from nuclear and wants to double it.
Chernobyl radiation is so low Ukraine loves nuclear energy and has enough to share with Germany.
What about Fukushima?
Fukushima
This map shows the radiation in Fukushima the year of the accident and one year after:
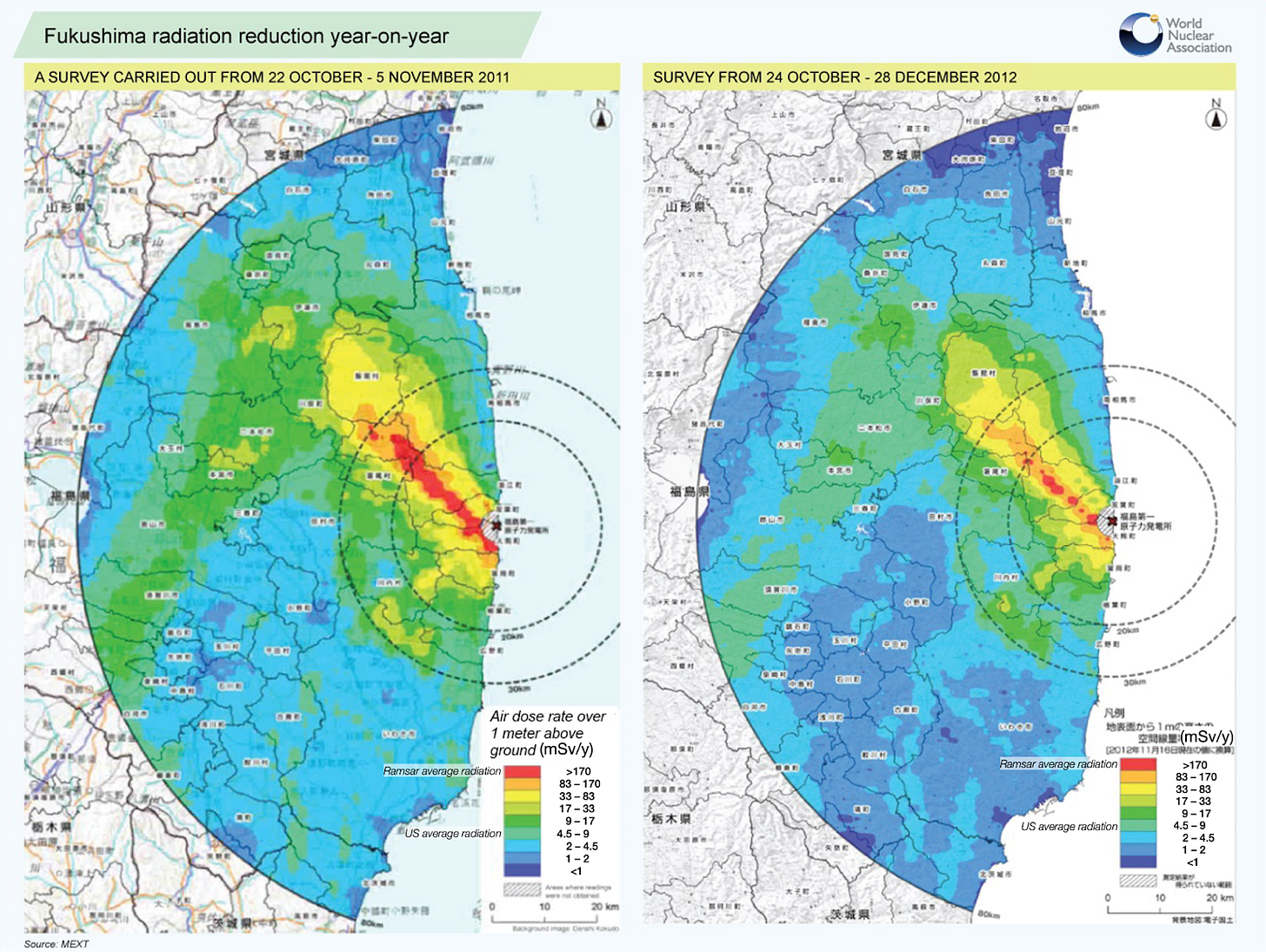
Radiation shrank fast. Within one year, most of the area had less radiation than what an American gets on average. Most of the area outside of the reactor had less radiation than what is natural in Ramsar, Iran.
If we look at an updated map:
Ten years later, the vast majority of the area has the same radiation as the average in the US, and nothing outside of the reactor itself has more radiation than can be found in Ramsar naturally.
Oh, by the way, to replace the energy produced by one Fukushima reactor, we would need the entire original exclusion zone covered with solar panels. Not the red piece, the entire thing.
What does all this mean?
It means that normal radiation from nuclear plants is nothing.
It means that even after terrible accidents, most of the area is still safe to live in, and quickly becomes safer over time.
It means that we’ve consistently overreacted about radiation.
It means that nuclear accidents are not great, but they’re tolerable.
Terrorism Is Nearly Impossible
If the worst accidents in history have had such a low impact, what could terrorists do? Maybe throw a plane at a nuclear reactor? But they’re protected by thick walls. What happens when a plane crashes against a big wall?
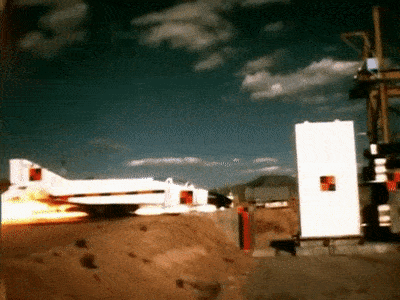
Nothing happens to the wall. The plane gets pulverized, though. The same would happen if a plane were to hit a nuclear plant.26
OK, let’s assume that terrorists get inside a nuclear reactor, close to the radioactive fuel, and detonate a bomb there. What happens?
If they’re extremely lucky and professional (how do they access the fuel?!), the fuel explodes, falls down, and that’s it. It remains contained in the facility. They would need to blow up the entire reactor and its containment enclosure for radioactive material to escape.
Even if they did, the radioactive material would be limited to the blast of a traditional bomb. Engineers in the control room could quickly stabilize the situation.
So why was Chernobyl so much worse than that? Because the fuel didn’t explode, it melted down.27 This heated up the water, steam pressure exploded, and the reactor exploded and created a plume of radioactive debris, which scattered in the atmosphere since the reactor was not housed in a containment building. This means terrorists can’t just blow up a nuclear reactor. They must take over the control room and the physical reactor and engineer a meltdown.
Engineering a meltdown used to be easier, because reactor designs were poor. But as we saw, new reactors have active and passive safety measures, including secondary containment. Bombing the reactor would not work. They would need to engineer a meltdown, stop all the active measures against it, and physically change the design of the plant. It would be easier to shut down the entire electric grid of a country like the US than engineer a nuclear meltdown.28 9/11 was child play compared to this. There is virtually nothing terrorists could do to create a new Chernobyl.
Nuclear Energy Does Not Mean Nuclear Weapons
Maybe your fear is not that terrorists blow up a nuclear reactor, but that a state with a nuclear plant uses it as cover to develop nuclear weapons. Indeed, there’s a huge track record of countries that publicly went for nuclear energy but privately aimed for nuclear weapons.29
But the overlap between “has nuclear energy” and “has nuclear weapons” is quite small:
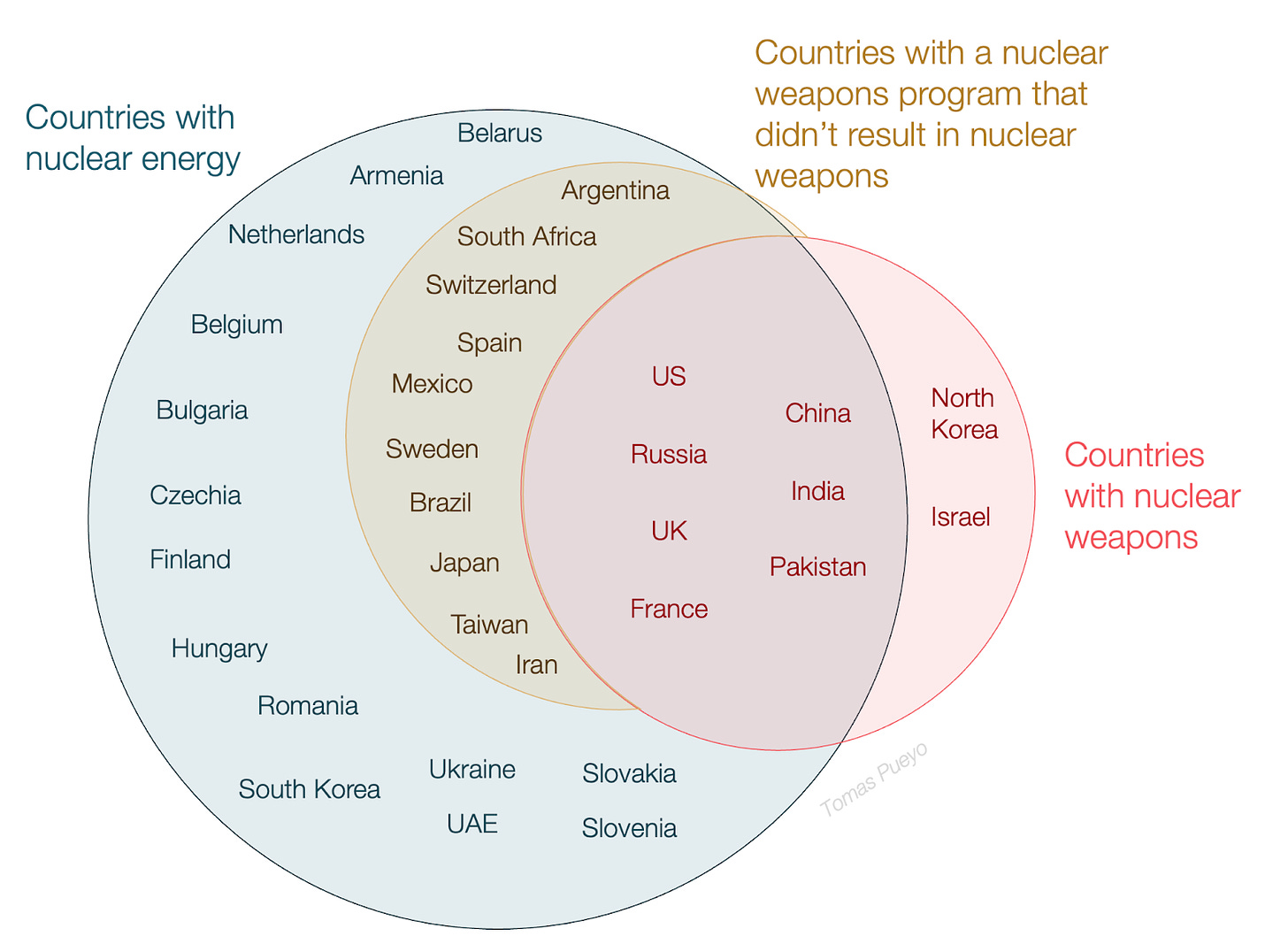
14 countries have nuclear energy but no nuclear weapons
2 countries have nuclear weapons but no nuclear energy. You clearly don’t need the energy for the weapons.
Most interestingly, 10 countries have nuclear energy and had a nuclear weapons program, but never developed a nuclear weapon. This shows how hard it is to develop nuclear weapons even if you have nuclear energy.
Iran is the perfect example: They have nuclear energy and allegedly have been pursuing nuclear weapons for over a decade, but they aren’t there yet. There are many obstacles, including:
It’s very hard to get your hands on uranium. Its trade is extremely controlled. Every gram is accounted for under international safeguards.
You also need pretty complex processes to go from nuclear energy fuel to weapons-grade. Nuclear fuel usually has ~5% fissile U-235,30 but must be enriched to 80% or more for a bomb. This enrichment is hard, expensive, and relatively easy to track.31
This is why the world knows when Iran has tried to get a nuclear weapon, and has prevented it from getting one so far32.
In summary for safety:
Nuclear energy has among the lowest deaths per energy produced, including bad accidents like Fukushima and Chernobyl—which, in reality, only killed 1 and up to 400 people respectively.33
A year living near a nuclear plant exposes you to less radiation than eating a banana or living close to a coal plant—whose pollution is much worse.
The odds of really bad accidents happening in the future keep falling. New designs are extremely safe.
When really bad accidents do occur, they’re much less catastrophic than we think.
Using a nuclear power plant for terrorism is virtually impossible.
Nuclear energy is neither necessary nor sufficient to develop nuclear weapons. Countries can develop nuclear weapons without the energy, and countries with the energy still have a very hard time developing nuclear weapons.
Nuclear energy is safe and clean of radiation.
Speaking of cleanness. What is the environmental footprint of nuclear energy?
2. Environmental Footprint
Nuclear energy:
Emits the least CO2 per unit of energy produced
Occupies the smallest land footprint
Produces very little radioactive waste
And the little radioactive waste it does produce can be effectively managed
Nuclear Emits the Least CO2
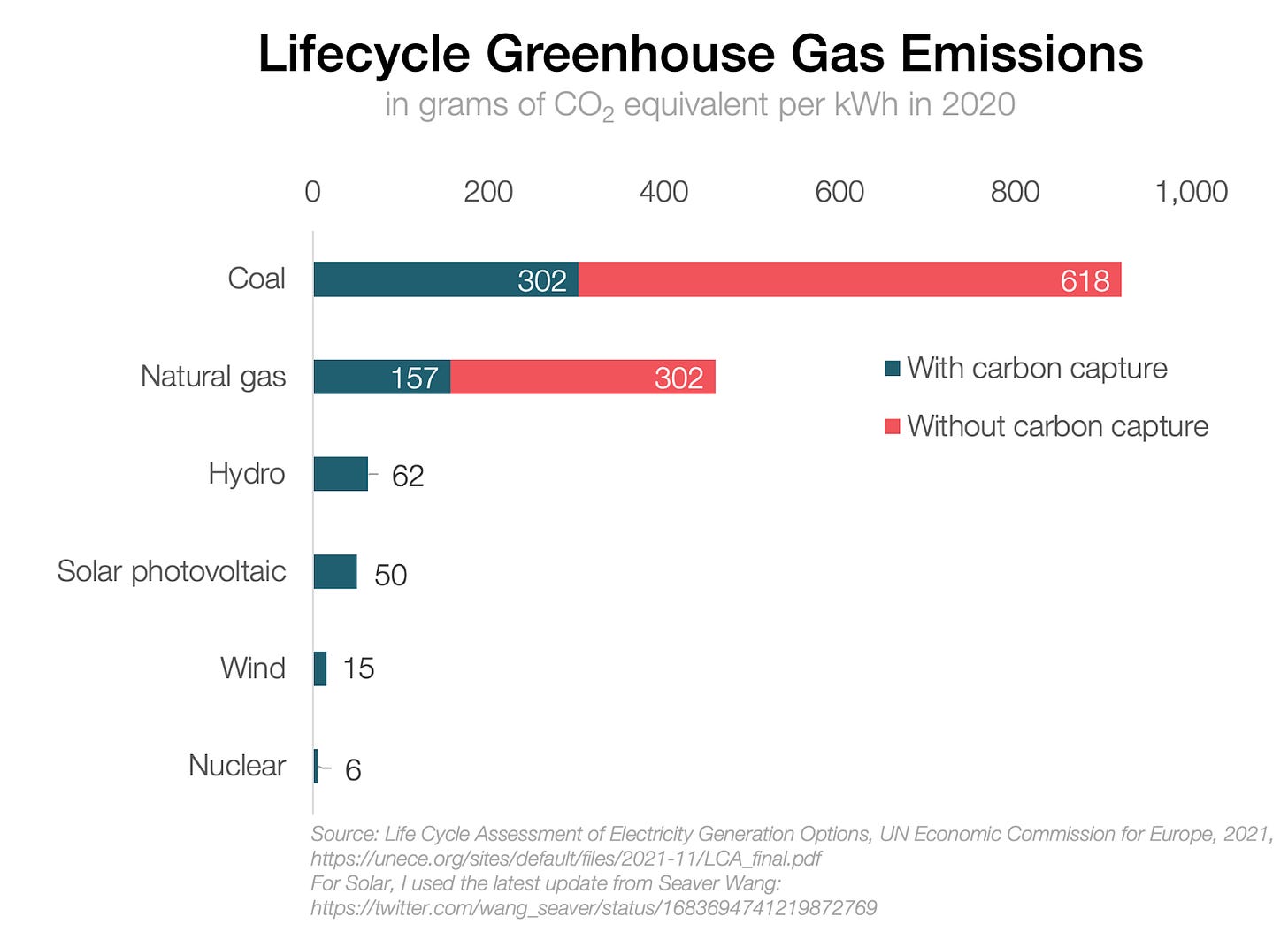
This chart includes all emissions throughout the lifecycle of the energy source, including production, construction, etc. What it doesn’t include, however, is batteries—needed for solar and wind to power the economy while the Sun doesn’t shine and the wind doesn’t blow. It also doesn’t include the emissions of electricity from fossil fuels to cover peak demand.
If we agree that climate change is the problem we’re trying to solve and that greenhouse gas emissions are its primary driver, then we should be shutting down coal and opening nuclear plants as fast as we can.
Nuclear Uses the Least Land
Nuclear uses between 40x and 400x less land than solar and 70x less than coal.34
Yes, solar uses a lot of space, and wind even more. Meeting all of the eastern United States’ energy needs might require 100,000 square miles of solar panels (an area greater than New England) or more than 800,000 square miles of onshore windmills (Alaska plus California), versus only a bit over 500 square miles of nuclear plants (the city of Phoenix, Arizona).35 Given the amount of real estate that solar and wind farms usurp, efforts to place them are running into entirely predictable local resistance, which will only increase as the easiest and cheapest sites are picked off.
Of course, technology improves, and solar panels will improve their efficiency. But most of the improvement is not in surface used: We can already transform about 25% of sunlight into electricity. We might be able to get to 40% or 50%, which would halve the area we need. But we can’t shrink it by a factor of 10. Until we get solar energy from space36, solar panels will use a tremendous amount of land.
Here’s an illustration to make the footprint of wind intuitive:
Here’s another:
We could power the entire planet with just ~15,000 nuclear power plants.37 To do the same with solar energy, it would take an area about the size of Argentina or India.
Nuclear Energy Generates the Least Waste
This might be counter-intuitive, as you might have grown up with the Simpsons hammering the problem of nuclear waste into your brain. But here’s what’s surprising about nuclear waste:
There is very little nuclear waste
Most of that nuclear waste is actually not that radioactive
The share that is really radioactive is extremely tiny
The amount of very radioactive waste will keep shrinking
It’s very easy to dispose of
There Is Very Little Nuclear Waste
How is this possible?
Radioactive uranium contains a lot of energy, so you don’t need much of it.
And nothing leaves the reactor except for electricity, heat, and spent fuel. The only waste is whatever has had contact with radioactive material, breaks down and needs to be replaced. Modern nuclear power plants last between 60 and 80 years… So far. They might well last a century or more.
Meanwhile, solar panels have a life of about 20-30 years, so they need to be replaced 2-5 times more than nuclear plants. And remember: The Sun only shines an equivalent of 6 full hours every day, so you need ~4x more solar panels to generate as much electricity as a nuclear power plant. And again, I’m not even counting the batteries here.
To produce the solar panels, we have to mine a lot of materials, including rare earth minerals. Also, some of its components are toxic and others are too expensive to recycle, so used solar panels are usually just dumped. It’s estimated that global solar panel waste will reach around 78 million metric tons by 2050—the equivalent of throwing away 60M Honda Civic cars.
Here, I’m picking on solar because it’s the next best energy after nuclear. But you can imagine the waste of something like coal is worse—and reaches us through the lungs, rather than being forgotten under a mountain.
For each unit of energy generated, nuclear needs among the least amount of material for construction, and the least amount for running. It has the least waste in terms of volume.
But how radioactive is that little waste?
Most Waste Is Not Very Radioactive
Nearly all the waste is low-level waste: not very radioactive. It comes from nuclear energy, but also industrial or medical uses. Some of this waste can be stored for a bit for radioactivity to decay before disposing. The rest can be simply buried like normal trash, maybe incinerating it first to reduce space.38
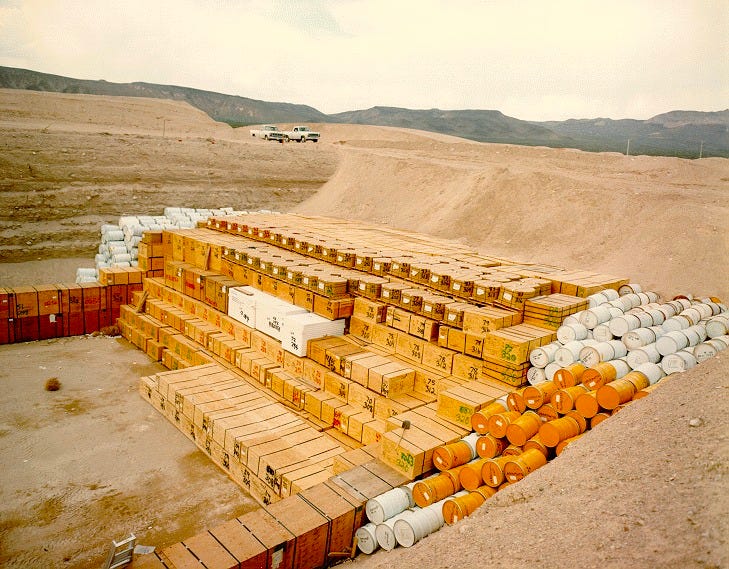
Some of it is intermediate-level waste. Most of it is managed like low-level waste but with more caution: For example, it’s placed in steel and / or concrete containers, and its radioactivity falls fast. Some of it is managed a bit like high-level waste.
How problematic is high-level waste?
There Is Very Little Highly Radioactive Waste, and We Know How to Manage It
3% of very little waste is very very little waste.
But why is it so bad? Because it’s still radioactive. Which means… it can be reused!
And that’s what we do. Since we started nuclear plants, about 30% of the fuel has been reprocessed, which reduces the volume of actual high-level waste (HLW) by 85%, and also dramatically reduces its radioactivity.39
If we wanted, we could create what are called breeder reactors40, which would need only 1% of the fuel current nuclear reactors consume, and would leave 100x less radioactive waste. Why don’t we make them? Because they’re a bit more complex, and there’s plenty of uranium to go around. Oh, and because waste is not a problem.
In the history of humanity, how much high-level waste have we generated? 50,000 m3. This is like a football pitch41, four meters high.
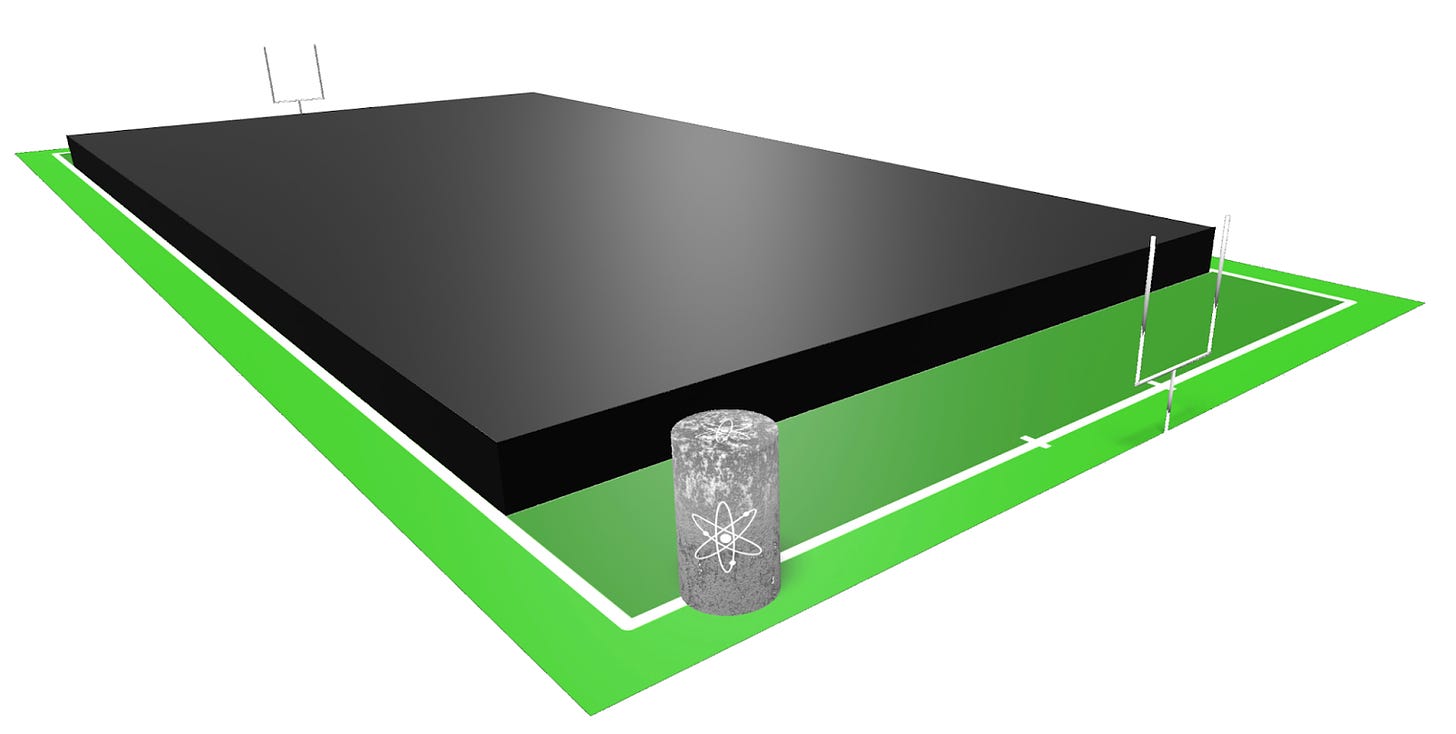
There’s so little HLW that most of it has been stored in pools on site so far, and we could keep doing this for a long, long time. It’s virtually nothing. Imagine this little waste to power our civilization! And again, this is fuel we could reuse in breeder reactors!
And even this HLW loses 99% of its radioactivity within 40-50 years!
So, if you wait for 50 years with the fuel in a pool, you can then store it 100x more safely.
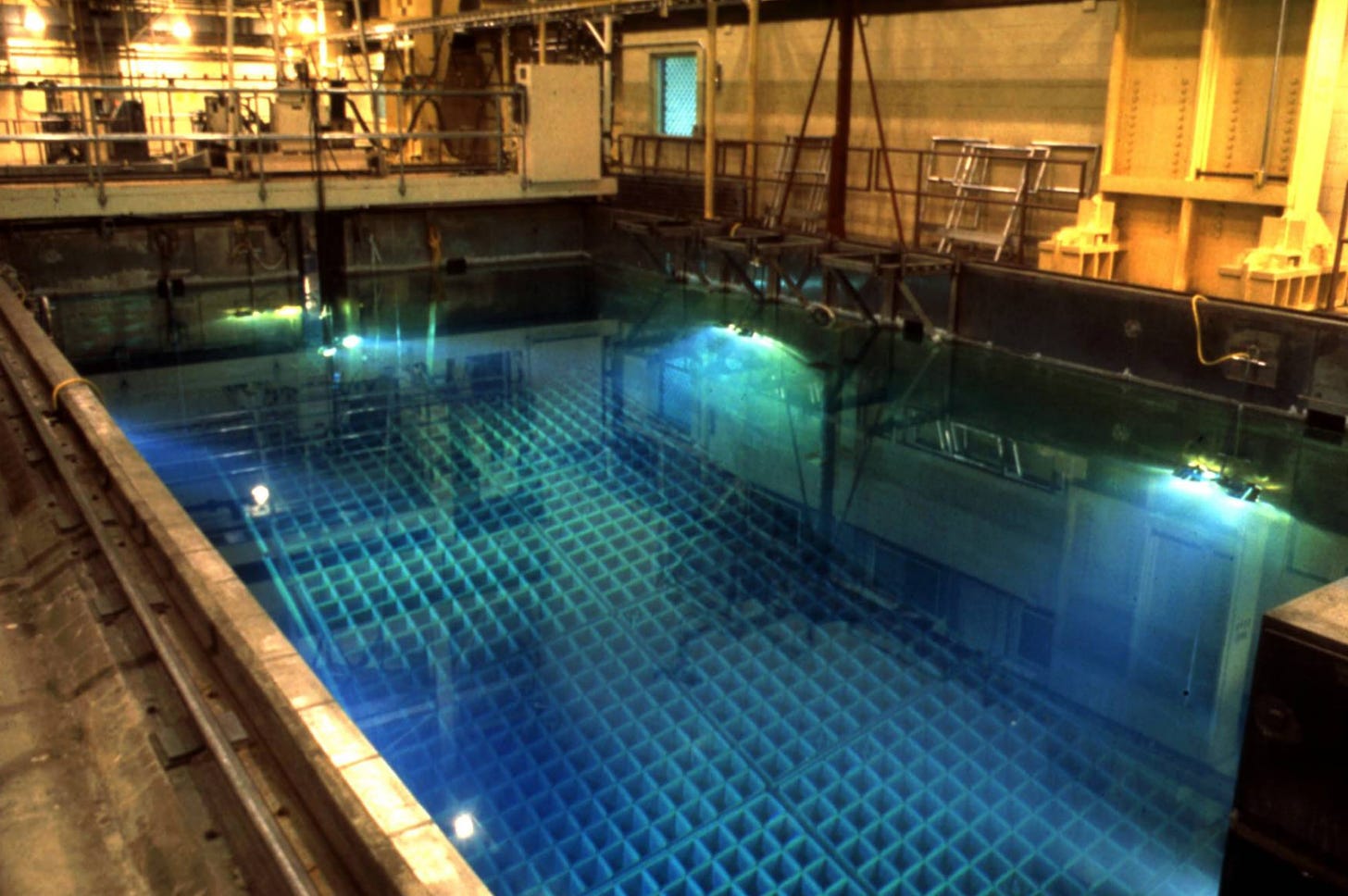
OK, even if there’s very little HLW in spent fuel, and most of it can be reprocessed, and in the future it will be reused completely, and it loses 99% of its radioactivity in 50 years, and we could leave it in pools for a very long time… Do we also want another solution for fuel that we don’t want to leave in pools? Yes, and we already have them. You can pack this fuel into steel, copper, and concrete, and you’re good to go.
And if you feel like you want extra security, do like the Finns and bury it underground in mountains that haven’t moved in billions of years:
I interviewed Mark Nelson here. Follow him for more nuclear info! I actually think that deep storage is a poor idea. It costs $1.33/MWh to do this, while the dry cask storage above costs $0.05/MWh and within 600 years radiation is so low you can handle the material with your bare hands. Then putting it so deep down makes it harder to withdraw for breeder reactors, and sends the message that such extreme measures are needed, when they aren’t.
Summarizing the environment section, nuclear energy is the most environmentally-friendly of all:
Emits way less CO2 than most other sources
Uses the least land
Generates the least waste, which can be managed to near zero, and we know how to safely dispose of what’s left.
But it would be useless to develop nuclear energy if the fuel was rare, right? Imagine finding out that we reached peak uranium like we’ve been worrying about peak oil for ages. Not a problem either.
3. Sustainability
Our sources of nuclear fuel are enough to last until the Sun engulfs the Earth.
Currently, we have found reserves worth 100 years of nuclear energy. Why only 100? Because why look for more when there’s plenty already?
If tomorrow we ramped up nuclear energy production and fueled the entire world with it, these reserves would only last us 6 years. The moment we needed to ramp that up, we would. How?
We can look harder
We can find uranium in granite
We can find uranium in seawater
We can use thorium instead
We can use breeder reactors
Very quickly, on each one of these options.
Look Harder
We’ve been concerned about peak oil for decades, and we never hit it. Why? Because the scarcer oil is, the harder we look for it, and the more we find. The same principle will be true for uranium, since we know there are trillions of tons of it in the crust. Uranium is a naturally occurring element with an average concentration of 2.8 parts per million in the Earth's crust. Traces of it occur almost everywhere. It is more abundant than gold, silver or mercury, and about the same as tin.
Use Granite
Remember the background radioactivity we talked about before? A lot of it comes from granite. In fact, granite-made houses with poor ventilation end up with reasonably high levels of radioactivity.
Every gram of granite has 50x more energy than a gram of coal.42
That’s because granite has some uranium in it, and that means it contains more energy than coal.
And as you can imagine, granite is abundant on Earth.
Use Seawater
There are 4 billion tons of uranium in seawater, which is about 500x the known quantities on land.
We can now pull 95% of the uranium from a seawater sample in just 2 h. And as we extract it, seawater will have an imbalance of uranium, so it will pull more of it from the seabed, making it virtually inexhaustible. We don’t do it yet just because it’s cheaper to mine it.
Use Thorium
It’s 4x more abundant than uranium, and at least 2x as dense in the crust, so it’s easier to mine. Thorium nuclear energy also produces even less waste and is harder to weaponize. As a result, there are many Thorium research reactors and even some companies aiming at commercializing it already.43
Use Breeder Reactors
I touched on this before: Breeder reactors use the radioactive uranium (U235) to make non-radioactive uranium (U238) radioactive. Since in spent fuel, 95% of uranium is 238 and only 1% is radioactive U235, you can multiply your fuel by ~140 this way.
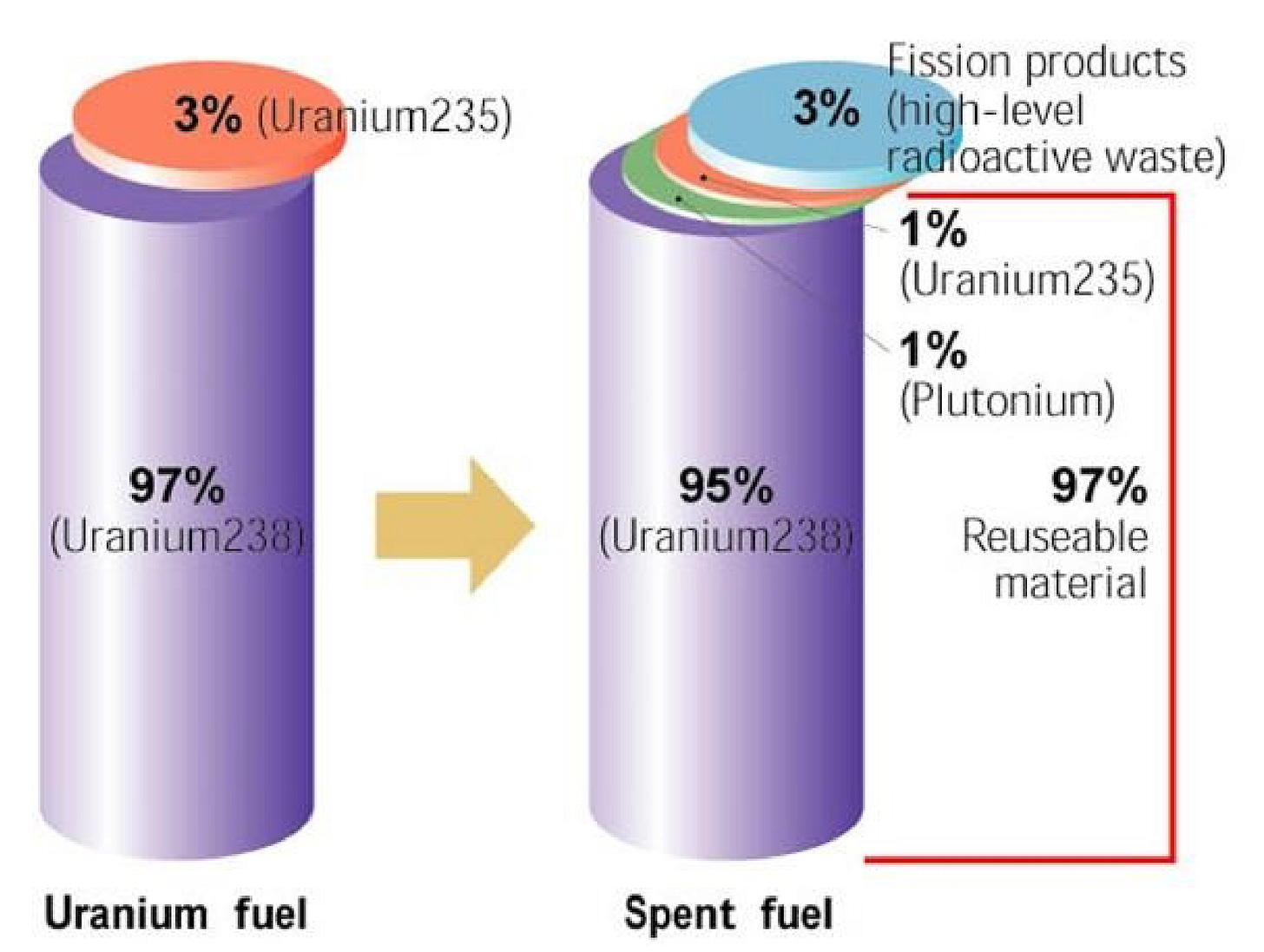
This is not a new idea. It’s been around since at least the 1940s, and the tech already works. It’s just not optimized because we haven’t needed to.44
In other words: There is more radioactive material than we can consume. By the time we finish it, we will have either long disappeared, or spread to the stars.
4. Reliability
Fuel Reliability
It would be dumb to go all in on nuclear energy, only to realize we are hostages to Saudi Arabia, Venezuela, or Russia again, like we have been in oil and gas.
Of course, the problem will disappear once we suck uranium from seawater. Or from granite. Or we use Thorium. Or we build breeder reactors. But even today, we’ve found uranium in so many places already!
And these are not unreliable countries either. Australia and Canada alone account for 38% of known reserves.
Today, Kazakhstan is the main producer of uranium.
But that would change quickly if Kazakh supply suddenly became unreliable.45
Always On, Predictable Supply
The lack or reliability of supply was always the biggest issue with oil and gas. Their biggest asset was that you could burn them whenever you want. Solar and wind power are limited by daylight hours and the wind blowing..
Nuclear has the best of both worlds.
We’ve been reliably producing nuclear energy 24/7/365 for over 50 years. France has generated over 70% of its electricity from nuclear for decades—the US, 20%.
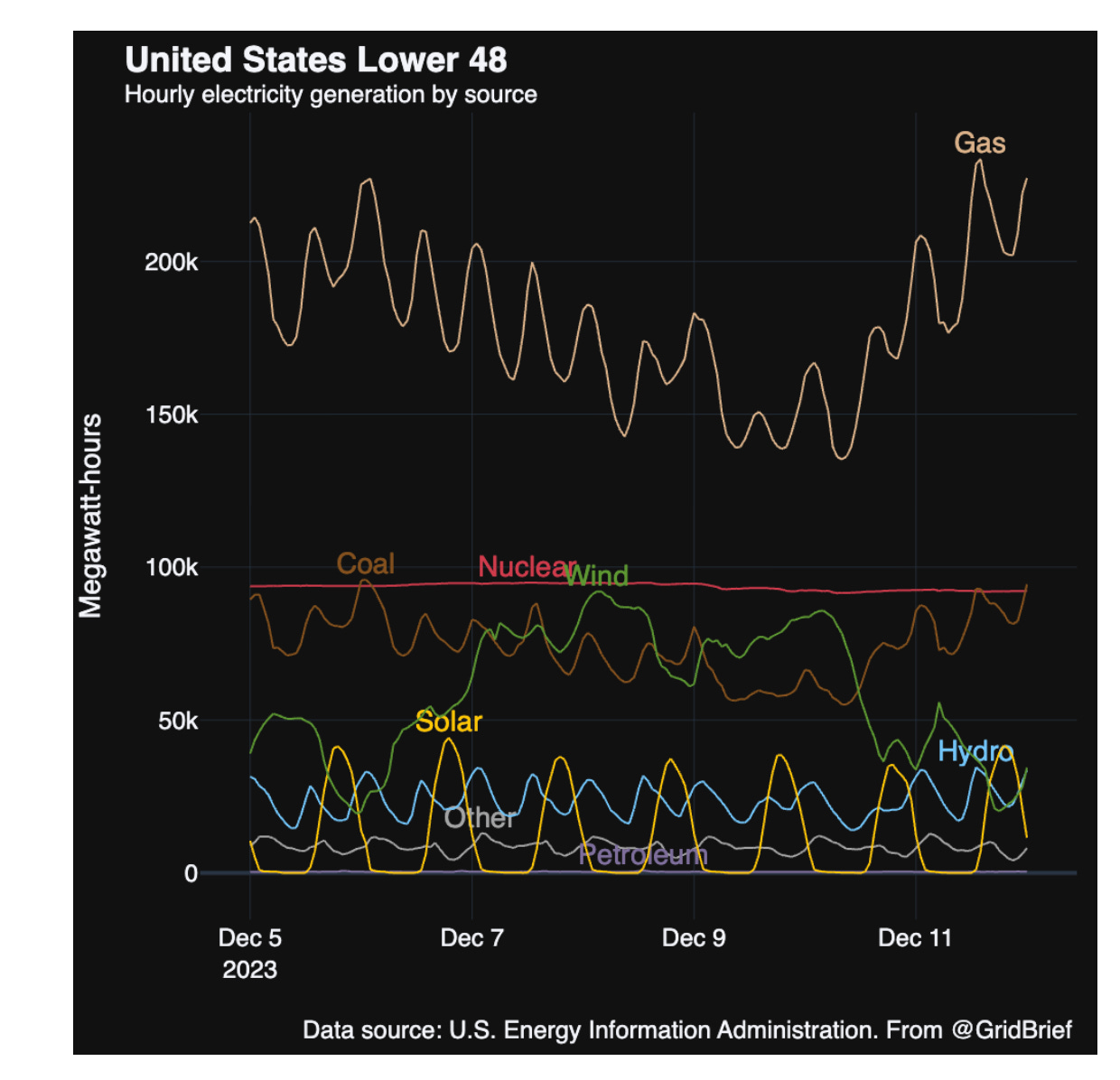
Supply Matches Demand
Up until now, nuclear energy was considered a baseload source of electricity: It was always on, at maximum capacity. As a result, it couldn’t increase production any further to face demand.
But that was by design!
Nuclear reactors are expensive to build (more on this later), but cheap to operate, so we have always wanted them to produce energy at maximum capacity. Shutting them down when demand was low would have meant we couldn’t amortize their fixed cost, so we simply didn’t build enough of them to cover peak demand. They were always meant to only cover base demand, and that’s why they were never designed to ramp up or down their electricity production quickly! It was a matter of design.46
But now that so many countries have realized the potential of nuclear energy to cover all electricity demand, they started changing their designs. Load-following nuclear power plants can ramp supply up or down fast—up to 5% per minute, so within 20 minutes they can go from shut down to full capacity.47 Nuclear reactors in France already operate this way.
Summarizing reliability. Nuclear is the only energy that at the same time:
Has reliable fuel sources
Produces energy reliably
Can ramp electricity generation up or down as needed.
It is the most reliable source of energy.
5. Politics
Supply Independence
When talking about the politics of energy, what you want is independence from any other country. I mentioned oil and gas: the dependence on the vagaries of the OPEC raising prices unilaterally, or Russia keeping you hostage in its war with Ukraine.
Would you rather be like Germany, Belarus, Hungary, or Ukraine, completely dependent on Russia for its energy? Or would you rather be like France, with almost complete electricity independence thanks to its nuclear plants, which produce 70% of its electricity?
This problem is also true of solar energy. China is dumping the price of its solar panels to corral the market. Indeed, 8 of the top 10 solar companies are Chinese. China controls 64% of polysilicon (the raw material used to make solar ingots and wafers) worldwide, its share of solar ingots and wafers is nearly 100%, its global share of solar cell manufacturing is 80%, and its share of refined rare earth metals is 90%. How much do we want to depend on China for our future energy needs? How could the Free World react to an invasion of Taiwan, or a takeover of the South China Sea if China controls the future supply of energy?
Since nuclear fuel is well spread around the world, much of it is in reliable countries, it can be extracted from the ocean, and the Free World controls the most advanced nuclear technology, relying on nuclear means energy independence.
It’s Easy to Store a Lot of Fuel
This is the US’s strategic petroleum reserve:
Notice how fast it went down in 2022 to fight inflation? Strategic reserves store lots of oil, but we consume so much, that if the US could no longer import oil, this reserve would last one month. This is how dependent the US is on other countries!
Why? Because oil is expensive and takes a lot of volume. 500M barrels would fill 32 Giza pyramids and cost $40B48!
But since nuclear fuel is a small portion of the cost of nuclear energy and is extremely dense, countries can store decades worth of supply. The US just decided to start that process in 2022, and so have countries like India and China.
Indeed, uranium has 100k times49 more energy per kilogram than oil, and uses about 2M times50 less space for the same energy. To get the energy equivalent of 500M barrels of oil, instead of 32 Giza pyramids, we would just need 40 m3. That’s about the size of a swimming pool!
As for costs, the average cost of a person’s annual energy, in fossil fuels, is $6,553. From nuclear energy, it’s $5.80: more than 100x cheaper!51 The US just allocated $75M for their strategic reserves of uranium. Compare it with the $40B just to buy the oil!
In other words: With nuclear energy, dependence on unreliable countries for fuel is over.
It Reduces Trade Deficits and Keeps Money Home
Since the biggest import in nuclear energy is the fuel, and it’s a small part of the cost, nuclear energy creates the least trade deficits. Countries can pay little money to foreign fuel providers, and get all the benefits of energy for themselves. This is how, for example, France exports electricity to all its neighbors.
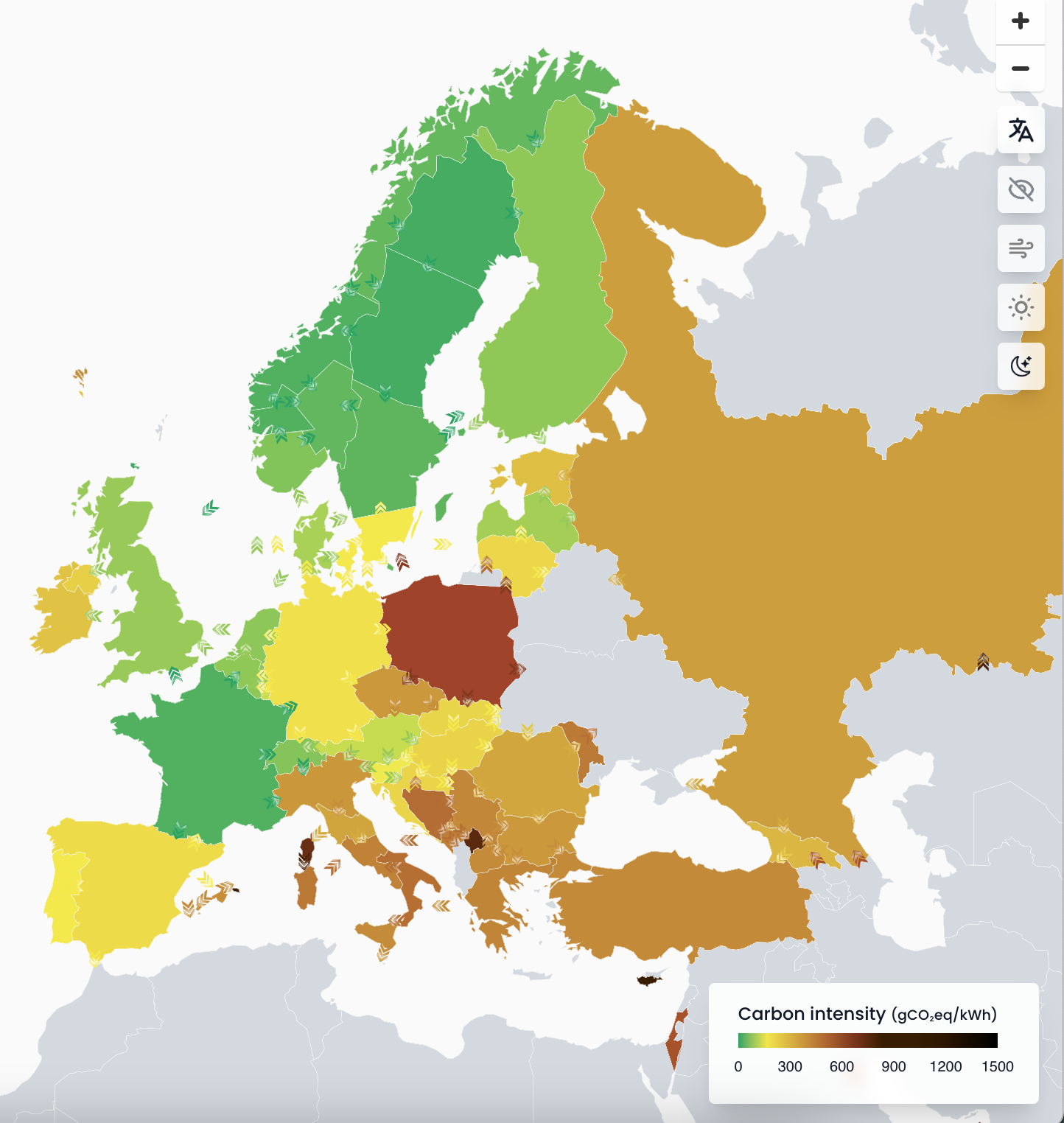
Countries bleeding money to buy energy could instead start making money by selling nuclear electricity.
You Can Build Them Nearly Anywhere and They Last Forever
You can only build windmills where there’s a lot of wind, and it’s preferable to build coal, oil, and gas plants close to mines, refineries, ports, or near pipelines. Hydropower is amazing, but limited because we can only get it in rainy mountain valleys.
In contrast, you can plant a nuclear reactor wherever you want. You just need some water for cooling. All countries can’t have all types of energy, but any country can get nuclear.
Price Stability
Many governments have suffered or have even been toppled because of high energy costs. We saw it in 2018 in the Yellow Vest movement in France, the Soviet Union in the late 1980s, the Arab Spring, the 1973 oil crisis, or Nigeria in 2012.52 A stable energy price is paramount for social and political stability.
Solar and wind, meanwhile, have haphazard production, and the more of them you install, the more they compete with each other and reduce the stability of the system, creating vast swings in prices every day and every season.
Nuclear energy brings price stability, since its fuel is such a small share of the total cost (~15%), and most of the cost is investment and operation, which can be done locally.
And nuclear brings that stability for decades, since it lasts so long.
In summary of political value, nuclear energy:
Eliminates dependence on unreliable countries
Allows for huge, cheap strategic reserves
Reduces trade deficits
Can be built by any country, anywhere
Guarantees the stability of electric costs
6. Economics
This brings us to our last point: the economics of nuclear energy. How expensive is it? Usually, energy costs are measured in LCOE—Levelized Cost Of Electricity—which is a dollar cost per megawatt-hour (MWh) of energy over the lifespan of an investment.
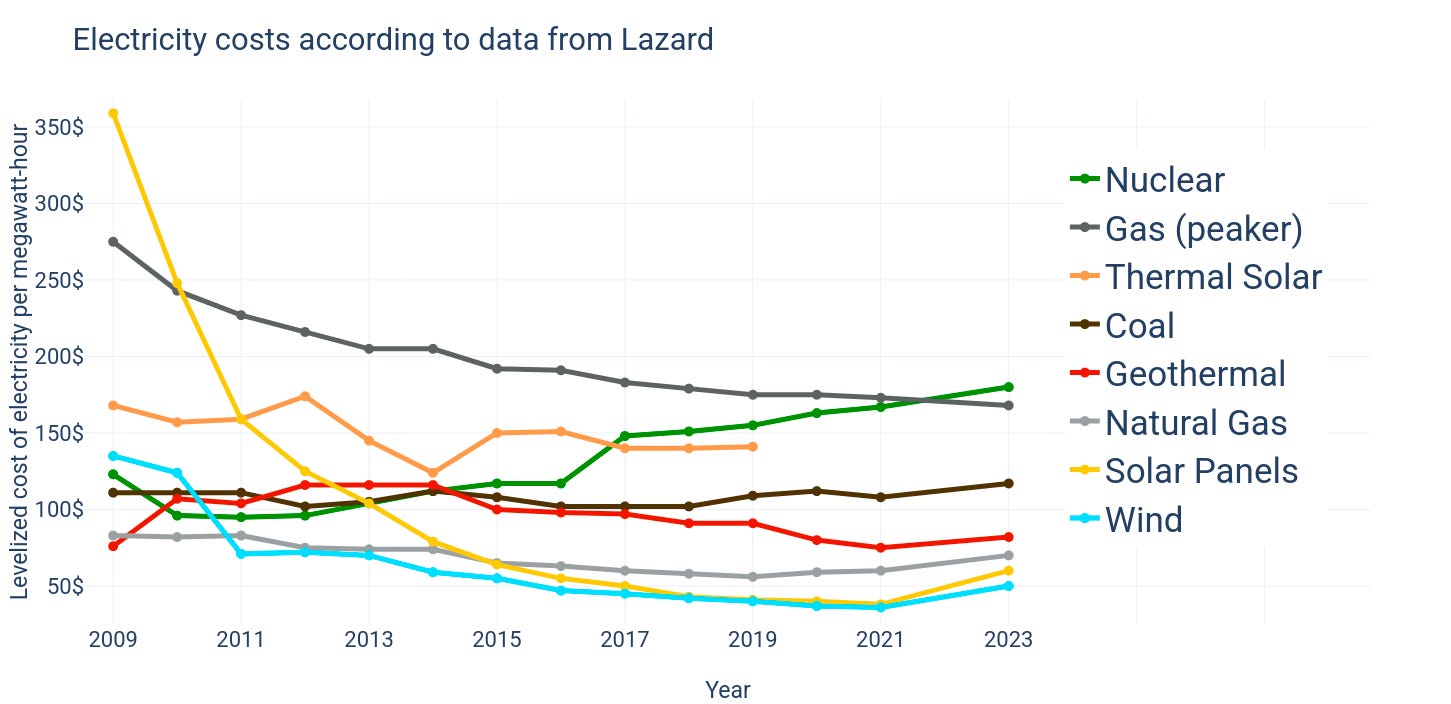
In the US, nuclear power is supposedly the most expensive source of electricity at about $180/MWh! Meanwhile, solar energy is at about $50/MWh, and coal in between. When you hear that “nuclear energy is too expensive”, this is what people are referring to. Now this data comes from Lazard, consistently quoted in the energy industry. But this reading can be misleading.
Look at an equivalent graph, but for South Korea instead of the US:
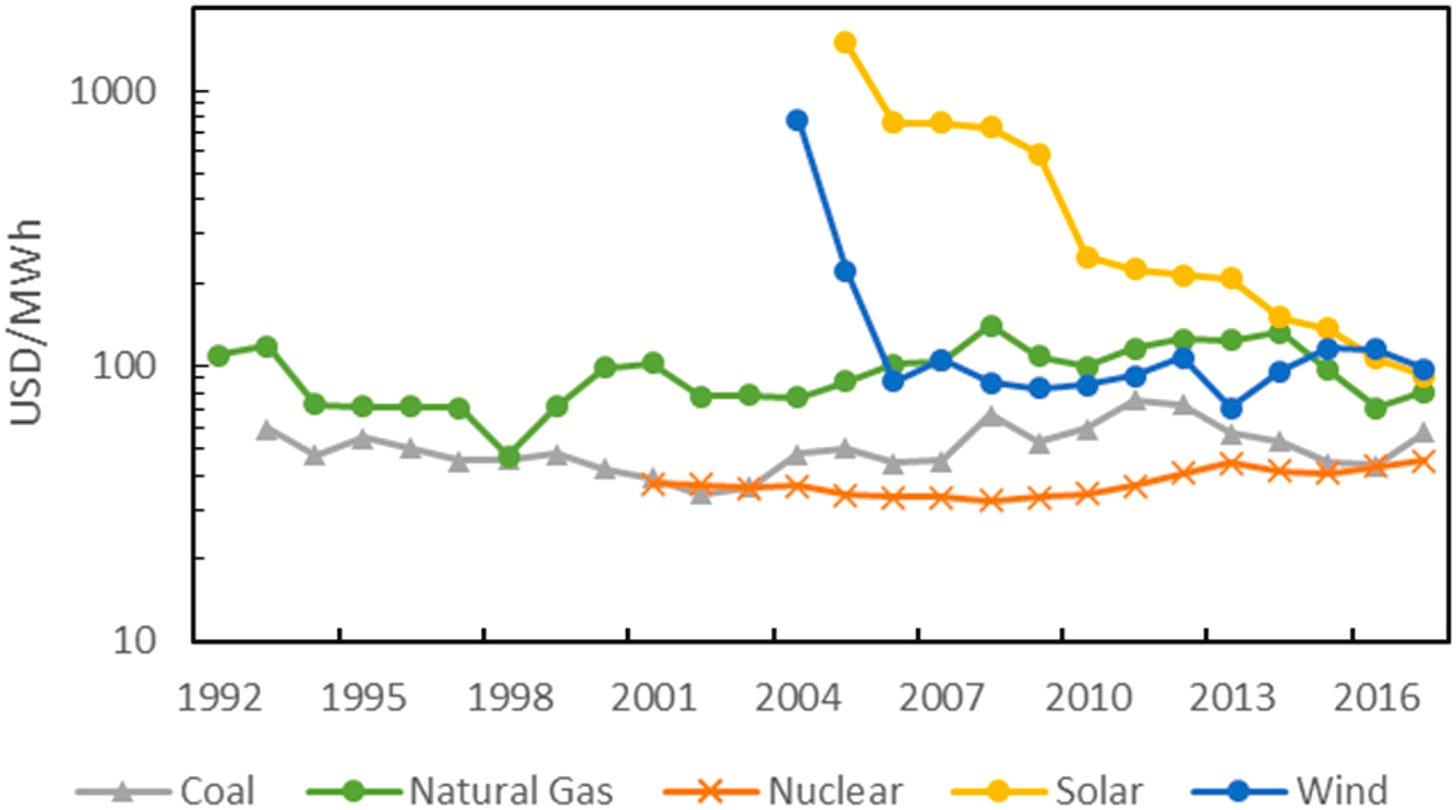
How come nuclear appears to be the most expensive source of energy in the US, but the cheapest in South Korea? How come nuclear costs have remained consistently flat in South Korea but have crept up for decades in the US?
Here is a similar graph comparing LCOE from another source:
Why such divergences? They’re caused by two types of issues:
Some calculations make assumptions that penalize nuclear energy, including subsidies, storage, CO2, cost of capital, operation years, and more.
The main cost of nuclear energy—building the reactors—is unnecessarily high in countries like the US.
6.1. Penalizing Assumptions
In the following sections, I take the projections from Lazard where nuclear appears so expensive, and break down all the ways in which it’s penalized.
Subsidies
Solar and wind are heavily subsidized by governments, especially the US.53
This is good for the long-term economy and environment, but it accelerates some technologies by making them look better than they actually are today.
Storage
Similarly, the biggest drawback of solar and wind is storage. This problem becomes worse the more we invest in them, as we see in California’s duck curve:
Every year Californians install more solar photovoltaic panels (from now on, just “solar”), the production of electricity increases during the day, with a peak in mid-day. So much so that the electricity price is now negative in the middle of some days, which means you have to pay to produce it! This only works if you invest to store that peak electricity. But that storage isn’t free. In fact, batteries are very expensive. So how much does electricity cost if you account for storage?
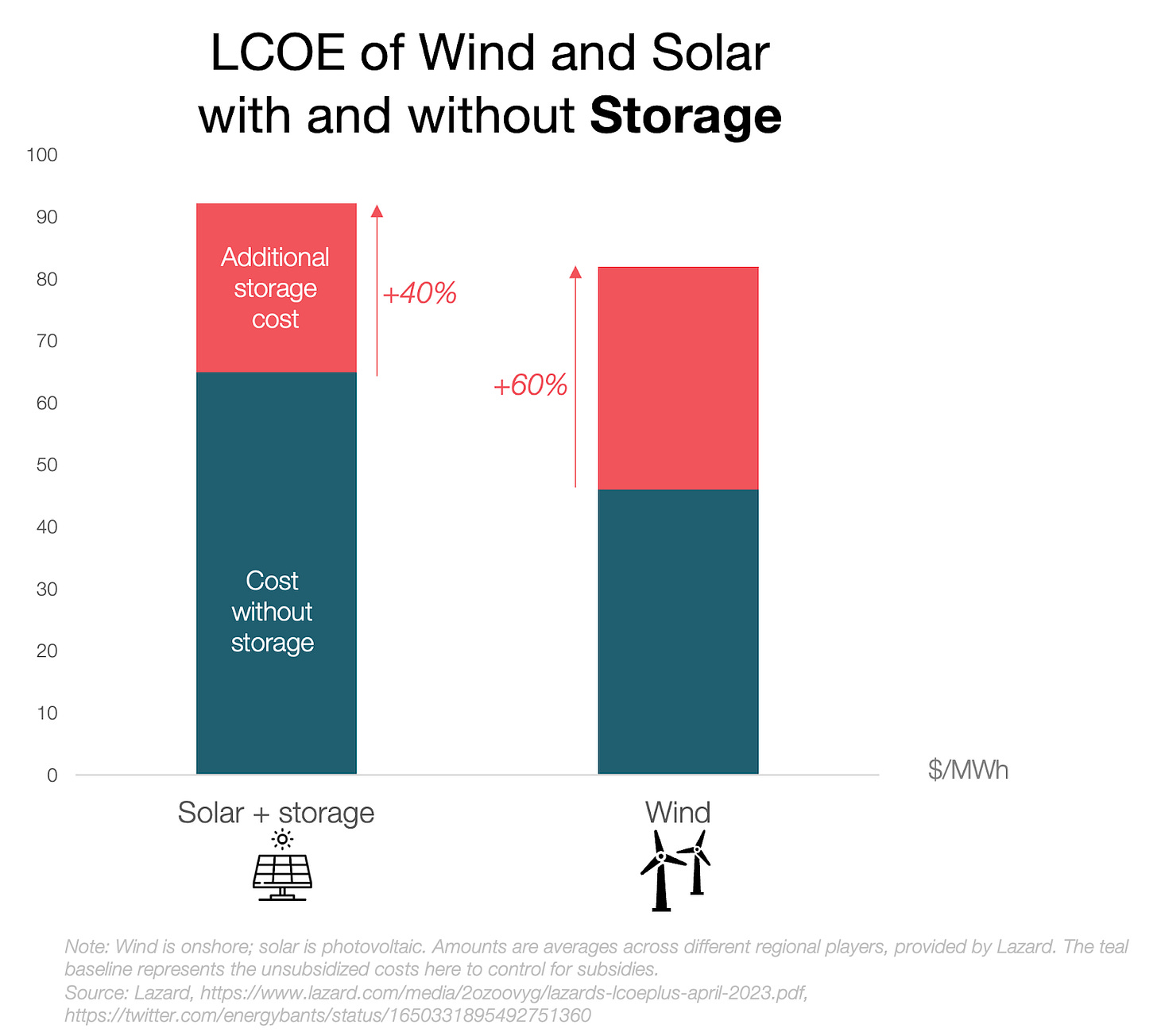
The more solar and wind costs fall, the more the storage costs will increase.
CO2
While wind and solar need storage and benefit from subsidies, fossil fuels of course emit CO2, but in the US that is not priced into the cost of energy. The price per ton of CO2 in Europe is $87/ton54. As we saw, the social cost of that ton of CO2 is about $185. But if we stick with $87, given the emissions produced by fossil fuels55, this adds up $86/MWh to the cost of coal and $30/MWh for the most efficient gas plants. Just this would make coal lose completely against nuclear, and raise gas to within striking distance of the high nuclear cost shown above.
Operation Years
Most models assume nuclear plants last 40 years. This matches what they’re licensed for. But this is not what happens in reality, and it’s extremely penalizing for nuclear!
Look what happens to the cash flow profile if you extend a nuclear plant’s life from 40 years to 60 or 80 years:
After 40 years, nuclear plants have paid back their loans. They are amortized. It’s all upside from there, and their electricity is dirt cheap. Why would we cut them then? We’re not doing it! In the US, the Department of Energy says 88 of 92 reactors that applied for an extension have been approved to go from 40 to 60 years of operation—and they don’t see a reason why they wouldn’t go to 8056. This is already a reality, but models keep assuming nuclear plants last 40 years! Look at France, enjoying 70% of its electricity from nuclear that was built and paid for decades ago. We should all try to be more like France.
If you put all these things together—subsidies, storage, CO2, cost of capital, operation years, or even some others57, nuclear becomes competitive, and frequently the cheapest source of electricity.
And we haven’t even tackled nuclear energy’s biggest issue: The cost of building plants! Let’s tackle that now.
Cost of Capital
The cost of nuclear power is extremely front-loaded: It takes a bunch of money and years to build the reactors, during which the plants don’t make any money. Only later do they start recouping that investment. This means that if the capital is very expensive, plant owners accrue interest for all these years before they can start paying back interest and principal, which makes them hyper sensitive to their cost of capital58. This interest rate is frequently very high, because of this long construction time, and because nuclear has a track record of delays and even plant cancellations.
But it makes a huge difference. Here’s what happens when the cost of debt goes from 10% to 5%:
Just with this change, nuclear becomes cheaper than gas! Look at how much its cost falls compared to other electricity sources! Imagine reducing this cost of capital further.
So there’s a triple whammy here:
Long construction times mean higher cost
They also mean more time to accrue interest
They also mean a higher interest rate, because the loans last longer and are riskier.
The most crucial question becomes: Why does it take so long and is it so expensive to build nuclear plants?
6.2. The Cost of Building
Here is the breakdown of energy costs, per cost type.
This clearly shows how expensive it is to build a nuclear power plant: The teal part is the biggest. But not only because it takes a long time and the cost of capital is high. There’s a thing called overnight cost, which assumes you could build a plant overnight. Nuclear energy is still expensive in those situations.
Nuclear power plants are not more expensive than coal plants with carbon capture. But they’re still more expensive than other sources, and on top of that, they take longer, are delayed more, and have a higher cost of capital when they’re built. Why?
Does this happen everywhere? If it does, maybe it's intrinsic to the tech.
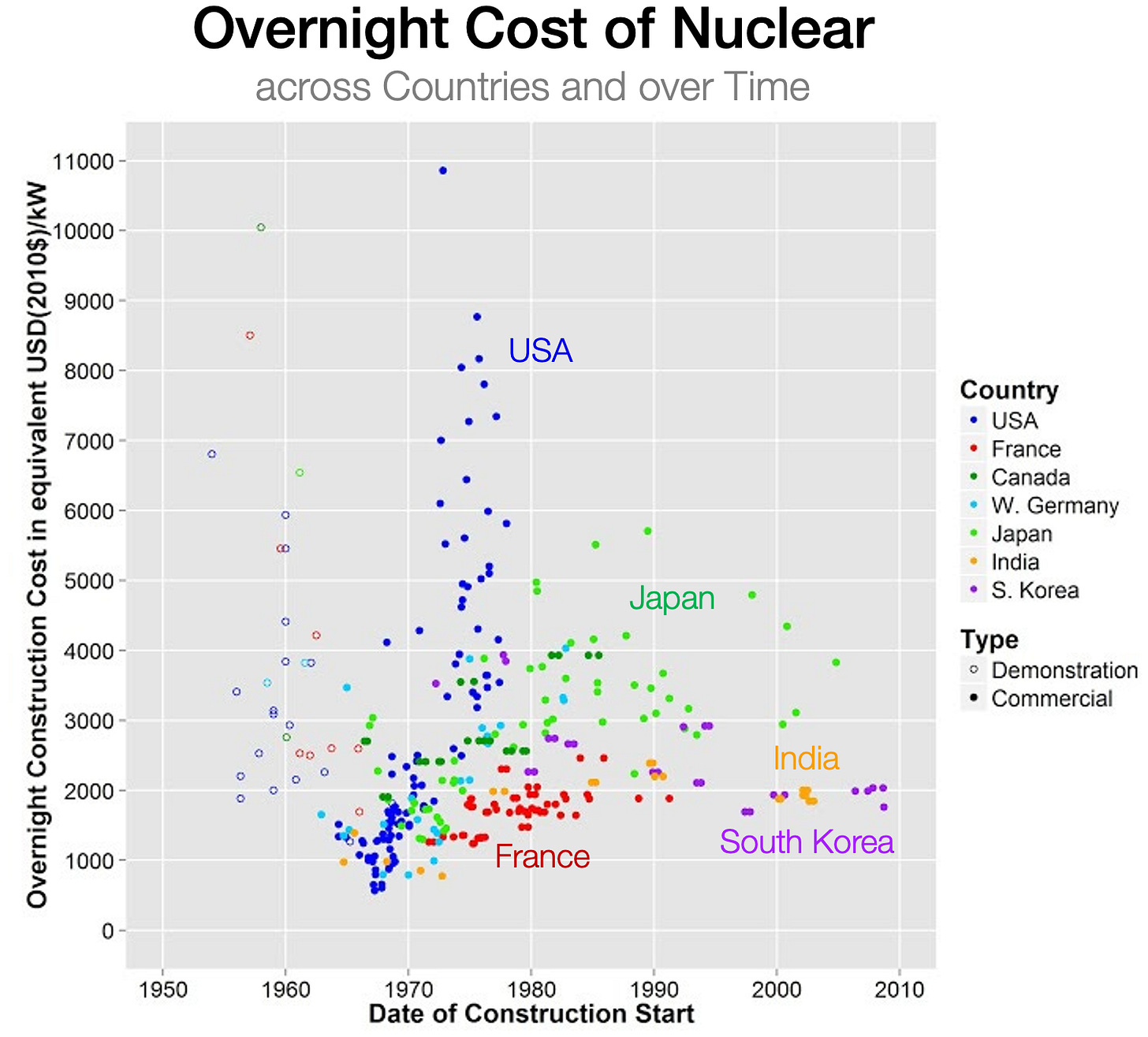
Aha! So France, South Korea, and India all built their nuclear power plants at $2000/kW, which is about the cost of a solar plant now.59
Nuclear energy doesn’t need to be so expensive!
Why does it cost 5x more to build a nuclear power plant in the US than in South Korea? This is the question.
Nuclear used to be as cheap in the US as in South Korea, and then for some reason costs exploded in the early 1970s, in a way that didn’t happen in most other places.
Around the same time, the US stopped building nuclear power plants:
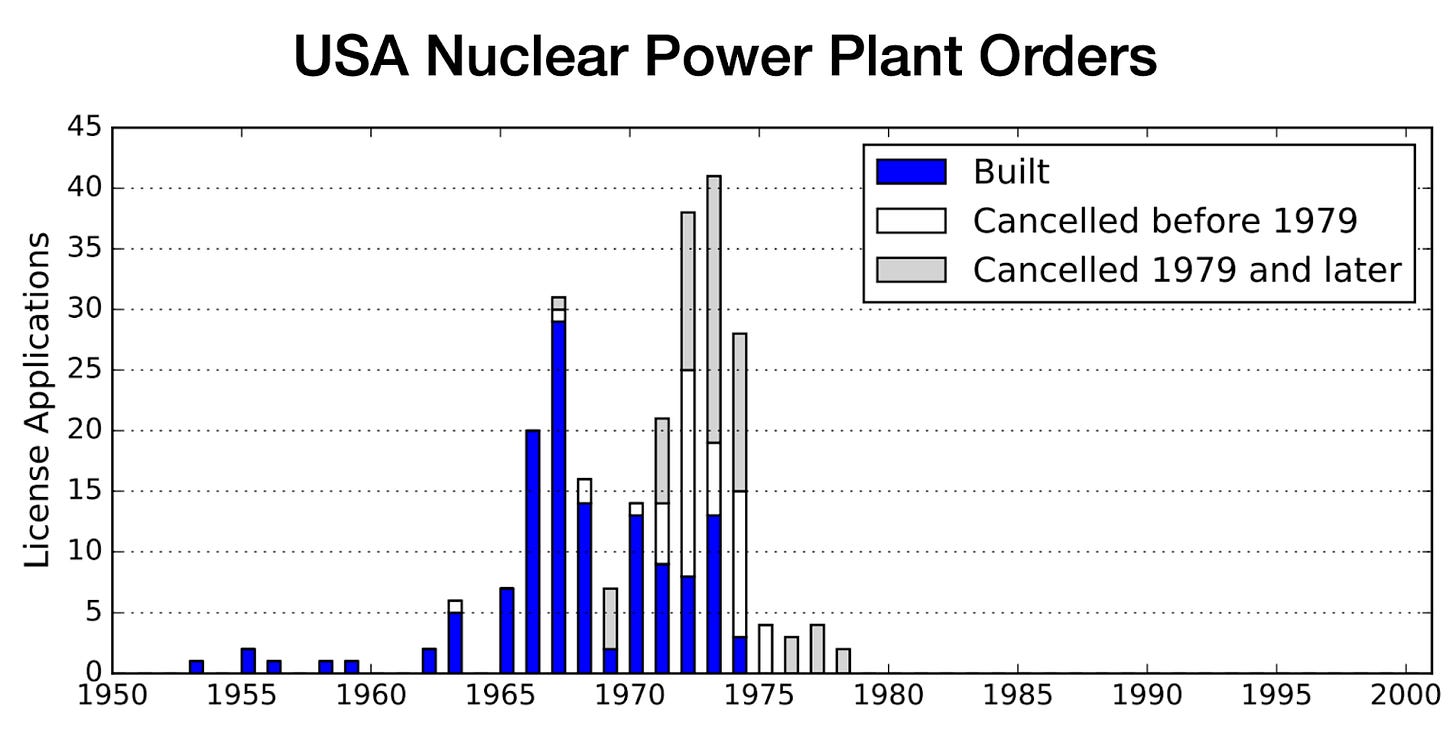
Around the end of the 1960s, construction time exploded too.
This is not the standard story. Japan has been building nuclear reactors in four years for 50 years:
Up until the end of the 1960s, every time the US built another plant, cost went down. We had decreasing costs thanks to learning! Like we do in every technology, and we’re seeing in solar and wind now. But at the end of the 1960s, we stopped learning, and it started costing more and more every year to build nuclear plants. It’s as if, instead of learning, we were unlearning.
What the heck happened in the early 1970s?60
Cost Explosion
This is how much more material was needed to build a plant in 1978 than 5 years earlier (to produce the same amount of energy):
How do you suddenly need twice as much steel for the same plant?
And it’s not just volume. The cost per unit also increased:
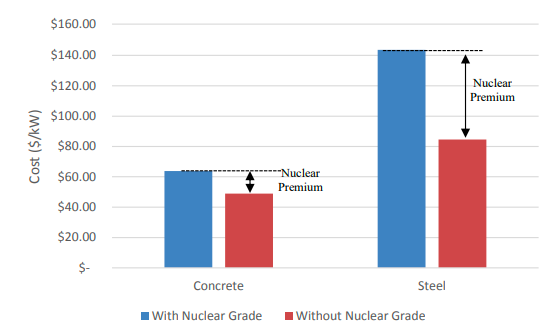
The very same concrete and steel is much more expensive for nuclear than coal. Why?
Every component is also more expensive—even the very same components as in other industries!
But it’s not just materials and components. What about human work?
The number of work hours spent to build a kW of capacity didn’t shrink like in most technologies where we learn. It grew by a factor of 5 for building (“craft”) and 7 for thinking (“engineering”).
This is the biggest source of cost increases:
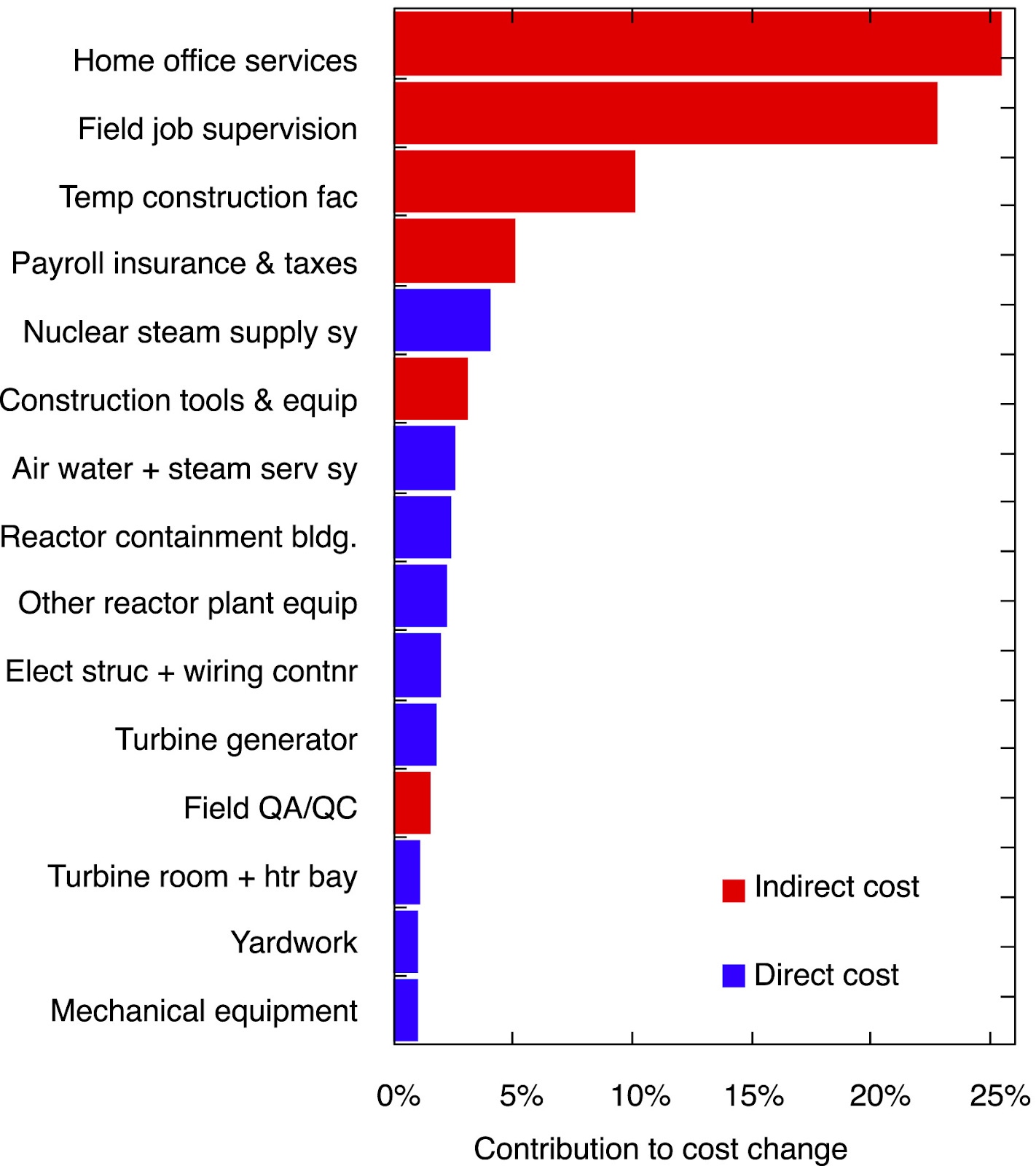
So what caused this huge and sudden cost increase?
Regulation
There was an explosion of new regulation. These are the federal laws on nuclear energy:
These new laws included creating new regulatory agencies
And these are the regulations, written not by Congress but by the nuclear regulators:
And not just new rules. The licensing process suddenly meant many more questions asked.
What happened?!
The Two Stories of Nuclear Building Cost Increases
There are two interpretations of what happened to increase regulation so much—and hence building costs.
One is that because regulators believed the effects of nuclear radioactivity were linear, therefore any radioactivity was bad, therefore they thought they had to limit radiation as much as possible.61
This is how you end up with less radiation close to a nuclear plant than eating a banana.
This is how you end up spending billions of dollars trying to clean up radiation to levels below the background.
This was institutionalized in a rule called ALARA: As Low As Reasonably Achievable. The goal was to reduce every single risk of radiation, which also meant reducing every single risk of accident.
ALARA meant that everything had to be triple thought, quadruple checked, have multiple independent systems to increase resilience… And to do that as much as is “reasonable”. And what is reasonable? On one side, we have risk reduction (always great!). On the other, we have… cost, which regulators were not incentivized to reduce. So “reasonable” meant “let’s reduce radioactivity until nuclear is barely economically viable”.
Regulators are not in the business of approving nuclear power plants. They’re in the business of reducing their risk. And in the process, they take $300 per hour of revision work. The more they revise, the more questions they have, and the more they keep revising, until enough millions have fed the machine to say “Yeah I’m sorry this is not good enough yet, try again next time. We’re from the government and we’re here to help.”
According to this narrative, this is not the only problem, because the bureaucrats look good when they get stamps, so that’s what they’re looking for. They go looking for documentation and more documentation to make sure everything is properly documented.
Instead, they could go to nuclear sites and check whether things actually work. This is how every industry does it. But no, that doesn’t work here. Testing whether things work in reality requires you to get out of your office, travel, get your hands dirty with testing equipment, work long hours… When you’re not accountable for things to work, better to check whether the procedure was followed on paper.
When you pay for paperwork, what you get is paperwork.
When you pay for results, what you get is results.
Another issue that arose is that, as all these new regulations were appearing, and all these new people were working to figure out everything that could go wrong, they would always find new ways things could break, so they updated the regulations to get nuclear plants to adapt. The result was that regulations changed across time, while people were building new plants. This made it impossible for nuclear developers to plan beforehand, and a massive amount of work was wasted waiting for changes and redoing work, ballooning costs.
With all this work, most companies decided to stop proposing new nuclear plants. This meant less competition, and hence, higher prices. It also meant less experience building plants, so a total elimination of the learning by doing that we see in other countries or industries.
This is one narrative. The other interpretation of these cost increases is that they were necessary. When nuclear energy was new, it was pretty cavalier about safety. The result was things like Three Mile Island and Chernobyl. But scientists and nuclear regulators caught this mostly on time in the West, and dramatically increased the investment in safety. Since so many reactors were being built at the time, they had to improve their designs at the same time as they were being built, resulting in massive time and cost overruns.
While coal and oil became cheaper in the 1980s, nuclear was still working through its security upgrades and wasn’t so competitive. So fewer reactors were started, which meant the industry started shrinking, many of the best professionals left it, and those remaining had no incentive in accelerating the construction of the last reactors, since they’d be out of a job when they’d finish them. This led to further time and cost overruns, and we end up with the last few reactors built in the US, France, and the UK taking more than 10 years to be built and multiple times more expensive than originally budgeted.
According to this narrative, one of the reasons of Japan’s quick reactor turnaround was that their safety was not as high as it should be—and hence Fukushima. South Korea was probably in the same boat.
I’m not sure which of these interpretations is true—maybe both have some truth? But the result is the same: We now have proven reactors62 that have passed all the regulations needed. Approvals are much faster now for these proven designs. The only thing we need to take advantage of that is to build a lot of them, so we can learn again how to do that quickly. This is why France recently increased its plan to build new nuclear reactors from 6 to 14.
With an end to regulatory changes, a new industry suddenly in growth, and the ability to learn with experience, we would:
Reduce waste while building
Learn how to build faster
Reduce overnight costs of reactors
Reduce the cost of capital due to shorter building times, less waste, and more governmental support
Shorter building times, smaller costs, and lower cost of capital would mean a dramatically lower cost of building nuclear.
This would mean much cheaper nuclear energy
This would mean more nuclear being built, and a continuation of this virtuous loop
And this is how you go back to the cost of nuclear energy being the cheapest source of electricity, like it used to be before all this insanity, or like it is today in South Korea.
Conclusion
Nuclear is the safest source of energy:
It has the least deaths per TWh produced.
It has few accidents.
When it does have accidents, few people die (except in Chernobyl, where up to 400 of them did).
Accidents don’t make huge swaths of land unlivable, only a tiny portion, and only for some time.
Terrorism is nearly impossible, and the risk not much worse than with other sources of energy.
Nuclear energy is not needed to develop nuclear weapons, and also doesn’t help that much.
Nuclear is the best source of electricity for the environment:
It emits the least CO2 per TWh produced.
It uses the least land.
It generates no pollution.
It generates little waste.
Most of that waste is not very radioactive at all.
The little that is radioactive is so little it can be kept in pools until we start reusing it as fuel. If we feel strongly about it we can always bury it.
Nuclear is the most dependable source of energy, and will last us until the end of the Earth. There’s plenty of uranium everywhere, and we’ve barely started looking for it. If needed, in the future we could get it from the sea, reuse 95% of the fuel which today we consider waste, or use Thorium.
Nuclear is the most reliable source of energy:
Lots of uranium comes from stable countries, and if needed, it could come from yet other places.
We can use it to produce electricity whenever we want.
Nuclear promotes political stability:
Fewer wars for resources.
Bigger strategic reserves.
More energy independence.
We can build nuclear plants nearly anywhere.
It reduces trade deficits.
It reduces energy price changes.
Nuclear can be the cheapest source of electricity:
It already is in some places, including South Korea. It’s quite cheap in other countries, like Japan and France.
The key is to reduce its building costs and time, which are so high in the US due mostly to the increase in safety regulation from the last few decades, and the industry’s resulting shrinkage.
The increases in cost due to regulation have been incurred and processed. Future plants should not be as expensive if we start ramping up the number of reactors we build.
We should also be realistic when making models: Account for storage costs or CO2, acknowledge that nuclear plants can work for over six decades, etc.
This will give us energy too cheap to meter.
Nuclear energy is the best.
If you found this article useful for others, share it with them and spread the idea that nuclear is indeed the best source of energy!
Thanks to Shoni and Heidi for your tireless editing.
Thank you Aydar Sarsembaev, Mark Whitwill, and especially Mark Nelson for your extensive help improving the article, and Alvar and Andy for your additional support.
When I started looking into nuclear energy, I was neutral about it. The more I studied it, the more I realized it’s the best current source of energy.
Fission to be precise.
The pledgers include United States, Armenia, Bulgaria, Canada, Croatia, Czech Republic, Finland, France, Ghana, Hungary, Jamaica, Japan, South Korea, Moldova, Mongolia, Morocco, Netherlands, Poland, Romania, Slovakia, Slovenia, Sweden, Ukraine, United Arab Emirates, and United Kingdom. Fun fact: These are not the countries building the most nuclear power right now. Those are China and India. I guess when you’re actually building you might not need to pledge. Or there are some politics involved.
And I’m not saying this as an anti-environmentalist. I’m green. I’m greener than lettuce. I’m so green I didn’t own a car for a decade. I’m so green I resisted buying one until my 4th child. My 4th broke me. I’m so green I write about cleaning up ocean plastic and practical solutions to climate change.
These deaths per TWh are fully loaded—that is, they include all deaths, from mining and installation, all the way to exhaust and pollution.
Dams breaking are not ancient news. The Saddle Dam accident in Laos in 2018 killed 71 people. The Kakhovka dam, in Ukraine, broke in 2023. Many dams are tricky, among other reasons because accumulated water undermines the foundations or even the soil beneath them in a way that is hard to even identify.
These work accidents are common in all sources of electricity. In fact, they are probably undercounted in nuclear, which would make it not the absolute safest source of energy, but just about as safe as wind and solar. That’s because, when people tally up deaths from nuclear, they tend to focus on nuclear fuel accidents, and not the routine, day-in-day-out deaths at work, like a piece falling on a builder during construction. AFAIK these are not counted anywhere, but an expert who spot-checked this assessed a standard number of construction deaths in nuclear. The reason why deaths are so much higher in sources like coal and gas is because these industries suffer many more deadly accidents, and they kill even more through pollution.
I’m basing these data points off of this article from Our World in Data.
For example, all nuclear reactors have materials to slow down the nuclear reaction (like boron) and materials to accelerate it (called “moderators”, like graphite). In Chernobyl, the endings of the boron rods were made of graphite! So when the rods got stuck, instead of slowing down the nuclear reaction, they accelerated it.
Nearly 90% of the Chernobyl deaths (the children’s cancers) would have been avoided if children had been quickly evacuated from the vicinity, rather than staying close to Chernobyl for days, consuming the radiated iodine that concentrated in milk and green leaves. When you study everything that happened there, all the mistakes they made, it is flabbergasting. It would make this article too long, so I won’t get into it, but research it if you’re interested. The HBO show Chernobyl only partially shows the dysfunctionality.
Three others developed leukemia or thyroid cancer. But 5,000 people work at the site daily, and 1.4 new cases of leukemia and 1.4 cases of thyroid cancer are diagnosed every year per 10,000 people. Assuming the workers are demographically representative of the population, that means you’d expect 1.4 such cancers per year (1.4+1.4=2.8 cancers per 10,000 people per year. Since it’s just 5,000 workers, that’s 1.4 cancers per plant per year). 10 years have passed since the Fukushima accident, which suggests a total of 14 baseline cancers. But we only saw 3 cancers, so it looks like Fukushima workers have 6 times less cancer than what you’d expect in the general population. Of course, if you’re managing a nuclear power plant, you might prefer to accept a death from nuclear radiation rather than have the family air its grievance in the media, so you have an incentive to say “yes, this is a nuclear-caused cancer, here take this payment and don’t talk too much about it”.
I wrote this from a plane, and I didn’t even realize until later. This is how unfazed we are about plane security.
I studied nuclear energy for my grad school engineering degree
Technically, that’s “enriched at 80% U-235 or more”.
Uranium ore has a concentration of ~0.7% U-235, so uranium for nuclear plants is just 5-7x more. Nothing like 130x the degree needed for bombs.
This is done with something called a moderator. Usually, it’s either graphite or water. When it’s water, it works at the same time as the moderator (to sustain the chain reaction), and the coolant (to extract heat to generate electricity). This becomes hard because the moderation effect can be more than the cooling effect, and a meltdown can ensue. This is why there are also control rods of something that absorbs neutrons and slows down the reaction. This is why I explained before that one of the many many faults in Chernobyl’s design was that the tips of the control rods were made of graphite (a moderator!!) and when the rods got stuck, the graphite was exposed to the nuclear fuel, moderating it (that is, accelerating the chain reaction) instead of slowing it down.
More details if you’re interested. It’s mostly xenon, cesium, and iodine.
The one reactor that melted down was closed, but there were three other reactors, which continued to operate for years. The last one closed only in 2000. Did you know that the last reactors were only shut down because of international pressure? Ukraine accepted to close the last reactors only because of international financing to finish other nuclear power plants.
Two Fukushima workers got 180 mSv of ionizing radiation. Apparently, symptoms of radiation poisoning start around 400 mSv in a short time (over 100x our baseline), and 4,000 mSv can be survived with prompt treatment. 8,000 mSv is fatal.
We also know that exercise delays aging, and AFAIK the process is similar; getting the body to believe it’s in attack mode and focusing on repairing DNA.
And this is true across the entire nuclear cycle. For populations living within 100 km of a mine and mill site, UNSCEAR estimated the population average individual effective dose at 0.025 mSv/y. The corresponding estimate for populations living within 50 km of a reprocessing plant was about 0.002 mSv/y. For nuclear power plants the average effective dose to populations living within 50 km of a plant was 0.1 µSv/y whereas the estimated effective dose to critical groups living within 1 km of the plant was 0.02 mSv/y. These effective doses to regional and local populations all correspond to less than 1% of the dose due to natural background radiation. The global average effective dose to the public from the nuclear power lifecycle also represents only 0.03% of the average dose to the public from all sources of artificial radiation.
A guy who was injected with plutonium (yeah…And he didn’t know about it! The type of thing people liked to do in the 1950s and 1960s… This is crazy, of course, and unconscionable. But we learned a great deal from it.) The plutonium stayed in his blood, and he survived for decades after being exposed to ~3.000 mSv/y! He died of heart failure when he was 79.
And amounts to about 90,000 mSv per year.
The plane, including its fuel tanks, collapsed against the outside of the 3.6 m-thick wall, penetrating a few centimeters. The engines were a better penetrator, but still dug in only 5 cm. The reinforced concrete outer walls surrounding a reactor are 1 meter thick, and there are many other thick steel, lead and concrete protection layers inside. This means that a plane crash straight into a nuclear reactor would barely make a dent.
The graphite moderator then caught fire, which heated the fuel up even further and accelerated its breakdown.
Terrorists could blow up transmission lines at several points in the electric backbone of the country. Since there are thousands of km of lines, they are not protected and are quite vulnerable. Dozens of attacks would be very easy. Much more so than taking over a control room and nuclear reactor against dozens of armed security guards with machine guns, and then apply enough knowledge to engineer the meltdown.
There are also nuclear submarines—those with nuclear propulsion. Some of them are designed to not need to refuel for their entire lives, over 30 years. Uranium is enriched at over 92-95% radioactive uranium. It would still not be able to explode in a nuclear bomb. Worst case scenario, it would also suffer from a steam explosion and escape of radioactive material, but very limited by seawater. In any case, this has more to do with nuclear weapons than energy.
5% is true for the most reactors in operation today, but for example Canada’s CANDU reactors use natural uranium (0.7% U235), and Encore Energy has already started enrichment of HALEU fuel (5-20%) for SMRs (Small Modular Reactors).
You might have heard about Iranian centrifuges. Iran has been pursuing a nuclear program since the 1980s and has claimed to have reached 60% enrichment.
Albeit maybe not for long since it claims it has reached 60% enrichment.
This includes an extremely conservative number of deaths from Chernobyl, and Fukushima’s deaths in the graph include the 2,300 deaths from the Fukushima evacuation. That said, most of the deaths caused by nuclear energy are likely due to standard construction, and might be comparable to wind and solar. We don’t know, because nobody keeps track of those deaths. I’m not sure why, but I hypothesize it’s because they think meltdown deaths are substantially more common than standard construction deaths.
Back of the envelope math to illustrate: A nuclear energy facility has a small area footprint, requiring about 1.3 square miles per 1,000 megawatts of installed capacity. This figure is based on the median land area of the 59 nuclear plant sites in the United States.
A wind farm would need an installed capacity between 1,900 megawatts (MW) and 2,800 MW to generate the same amount of electricity in a year as a 1,000-MW nuclear energy facility. Such a facility would require between 260 square miles and 360 square miles of land. That’s a ratio of 200-270x.
A solar PV facility must have an installed capacity of 3,300 MW and 5,400 MW to match a 1,000-MW nuclear facility’s output, requiring between 45 and 75 square miles. That’s a ratio of 35-57x.
But then the nuclear sites don’t have just one reactor. They have many (92 in total in the US). And usually, the amount of land they own is much higher than they need in case they want to expand the plant. That space allows for 2-3x the number of reactors built. New nuclear reactors can also produce more than 1 GW. Taking real examples like Diablo Canyon Nuclear Plant vs the Topaz solar plant yields a result of 379x more energy generated per km2.
According to congressional testimony by Armond Cohen of the Clean Air Task Force.
Or from the sea, which would be very cool.
A typical plant can have a power of around 1.2 GW of power, which yields an energy of 1.2*8760 = 10,512 GWh per year. The global annual energy consumption is 580 million terajoules, which is equivalent to 160,000 TWh. Therefore, all world energy could be obtained by 160,000,000 GWh/10,512 GWh so ~15,200 nuclear plants.
The radioactivity released by the burning is close to zero since the stuff is not very radioactive to begin with, and I believe most of the radioactive elements are very heavy and fall as ash. They aren’t converted into smoke.
The remaining fuel would only take 9,000 years to become as radioactive as the background.
There are two types of uranium: The U238 isotope, which is stable, and the radioactive uranium, U235. The vast majority of uranium is U238. We power our reactors with U235. But what we could do is use the chain reactions of U235 to hit the U238 and make it radioactive. This way, we would burn not just the U235 but also the U238.
This works whether you use the term “football” for actual football or the American handball version. We’re talking about orders of magnitude here.
With 1950s tech, we could get 20% of the energy contained in granite, which still means 10x the energy per ton compared to coal.
Somebody has tested plugging thorium and uranium into CANDU breeder reactors, and the Clean Core Thorium Energy company is exploring its commercialization. India is a leader in thorium research.
It will likely become economically viable in the 2nd half of the 21st century.
Canada for example could easily ramp up production, but doesn’t because it’s not economically worth it right now.
Indeed, boosting or decreasing production is conceptually as easy as speeding up (with moderators like graphite) or slowing down (with boron, for example) the chain reaction.
The concept of flexibility to increase or decrease production is called “dispatchability”.
At December 2023 prices
U235 has 2M times more energy per kilogram than oil. If we assume enrichment at 5%, that’s 100k times more energy than oil. If, however, we had breeder reactors, we would be back to 2M times more energy per kg.
From Where Is My Flying Car? by J. Storrs Hall (2021).
The Yellow Vest movement was in response to an increase in fuel taxes to fight climate change (fuel for the car is still needed and independently priced from electricity, since most cars in France are not electric yet. France could only have issues with energy prices where its nuclear power doesn’t reach). The Soviet Union suffered a big budget hole because of low oil and gas prices, the Arab Spring was caused among other reasons by high energy, fertilizer, and food costs, all connected. After the 1973 oil crisis, leaders in countries like the US or the UK were not reelected.
Note the US just approved heavy subsidies for new nuclear, but they have not been used much yet, and the costs shown here don’t reflect these subsidies.
0.986 t CO2 eq/MWh for coal-fired plants, 0.486 t CO2 eq /MWh for gas-turbine plants, 0.352 t CO2 eq /MWh for co-generation & combined-cycle plants, in France, according to this.
Many early designs didn’t reach 40 years. That’s what happens with new technologies. Of course we learned from that, and all designs are now expected to last at least 40 years, but are designed for at least 60, and if the track record of existing plants is a good enough indication, 80 years or 100 sounds very reasonable.
For example, nuclear plants are being explored as a source of heat for nearby populations, which would reduce the need for other sources to heat them. For example, we mentioned land, but not the concept that the more land wind and solar use, the more people will dislike that, and be sensitized to it, making it into an externality that we’ll have to start pricing. Or simply, more energy construction can increase the price of land for this type of use, which is not something modeled so far. I didn’t include the cost of all the non-CO2 pollution that coal produces either. Etc.
You can imagine “cost of capital” as the interest in loans. It’s not just that as it also includes the desired return to equity investors, but conceptually it’s the same: The money back that people want to finance the operation. Throughout the article I tend to use interest rates and cost of capital somewhat equivalently because in the grand scheme of things it doesn’t matter, the same principle is true for debt and equity: The riskier the investment, the more expensive both will be, and if the state intervenes, it can bring down the cost to at least its own cost of capital, which is the interest rates it pays on its own debt.
France is now more expensive, but it has only built one reactor in the last few decades, and its problems are connected to what we will see about the US in a moment.
All of this happened well before Chernobyl, and even Three Mile Island.
Westinghouse’s AP1000 is probably the equivalent of the Boeing 747 for nuclear reactors. The underlying technology, PWR or Pressurized Water Reactors, has never killed people in a reactor meltdown. Chernobyl was an RMBK and Fukushima a BWR (Boiling Water Reactor). The AP1000 uses 80% less concrete and steel than previous PWR generations.
Thanks for this very detailed article.
I feel you have missed some things, though, mostly about the operational part.
"Nuclear doesn't need to be that expensive", but it is. It also takes a very long time to build a plant. And it can only be switched on once it's finished (unlike solar, where you can switch on one row of panels at a time).
We have the recent example of Olkiluoto 3, in Finland. The contractor went bankrupt. In Vogtle, in the US, the contractor, Westinghouse, went bankrupt. You point to South Korea, which is true, they have the industry knowledge, they build the same type of reactor all the time. But for instance, Europe is not South Korea. I analyse in further detail in this article the last 20 years of nuclear building in Europe: https://europeanperspective.substack.com/p/schedules-costs-and-risks-of-new
"We don't need to depend on dubious countries", but we do. Nuclear plants in Eastern Europe, which have been built under a soviet design, need soviet fuel and they had to buy it from Russia. It's been very difficult for western suppliers to provide fuel (not sure if that's solved now).
On top of that, most of the nuclear knowledge in the world comes from Russia, from the national company Rosatom. Rosatom is building nuclear in Turkey, are we OK with this? Look at Finland, they cancelled one plant in progress because 30% of the ownership was Russian.
Missing Rosatom and Russia's role is one of the main shortcomings I find in this article.
"We could build wherever", but we can't. You still need to find a location as far as possible from fault lines and possible earthquake-risk areas.
All in all, if I had a lot of money to invest, in Europe, and I wanted to generate electricity, I would not go for an option in which the continent industry doesn't have experience, or good track record, and that it would take many years to generate the first Kw (if it ever does).
Plus, decommissioning plants, which needs to be done, and it's extremely expensive and time-consuming. Calder Hall in the UK will be decommissioned one hundred years for now (if things go according to plan).
You completely missed a crucial aspect of reliability: how often nuclear plants are offline. This matters a lot when your grid relies on them and you don't (and really can't, practically) have a lot of redundancy. In the last few years, France's reactors have famously been offline for crucial months even as energy crises have ravaged Europe, throwing the electricity sector in the rest of Western Europe into chaos. Here in Sweden, we have four operational reactors and two of them were offline last week, during an unseasonable cold-snap. Electricity prices shot up 7x overnight. Even Finland's brand-new nuclear plant (TWO DECADES in the making and billions over budget) has been offline many times this year, again, shooting up prices.
People love to talk about how renewables are unreliable, but the inconvenient truth is that *all* power sources are unreliable. Including (and especially) fossil fuel generator plants. And nuclear is no exception. And the problem with nuclear power, especially, is that it is so expensive and capital-intensive to build and uneconomical to just switch on and off, so you aren't going to build more than your baseline load. So, when your reactor's down, you don't have a backup. When dozens are down (as in the case of France recently), you are in real trouble.
Nuclear power is no panacea: It take a very long time to construct, it's extremely challenging to finance, it requires a lot of complicated maintenance even to keep running (much less run with optimal safety), and it is vulnerable to extreme weather, too.